The year is 2021, almost 5 years after the signing of the Paris climate agreement, an ambitious accord to limit global warming to 1.5°C. In the meantime the earth has already warmed up more than 1.1°C compared to the ‘pre-industrial’ era (1850-1900).[1] And so far, this curve does not show any signs of flattening any time soon. The fight for socialism is the fight for the future, and thus also the fight against climate change. But how do we fight climate change effectively? In this article I will argue that limiting this battle to the use of solar panels and wind turbines, which is the predominant strategy across the entire left, is wholly insufficient and completely unrealistic. I therefore advocate to complement this strategy with the use of other energy sources, including nuclear fission energy.
1. Why solar and wind energy is insufficient
Development and limitations of solar panels and wind turbines
I will start this first part by analysing both the current state of solar and wind technologies, and how we can expect these technologies to improve in the future. I will continue with talking about the storage of electricity. And conclude with arguing for an electricity grid which is as diverse as possible.
The not-so-unlimited potential of Silicon
The efficiency of silicon solar cells has been steadily increasing over the years. However, it would be incorrect to assume that this trend will continue on in the future. All good things must come to an end, and as all too often thermodynamics is the reason we can’t have nice things.
The efficiency of any solar cell is thermodynamically limited by the Shockley-Queisser limit,[2] for Silicon solar cells (read: all commercially available solar cells) this happens to be 29.43%[3]. Of course nothing is perfect, and in practice the actual efficiency is always lower than this limit. Modern commercially available solar cells typically have an efficiency of around 24%, and thus are already very close to their fundamental limit.[3] This is why the developments in the efficiency of solar cells is stalling.
So how do we continue improving the solar cell? Luckily, it is still possible to reach higher efficiencies, we just have to choose a different material! Independently of the chosen material the maximum efficiency has been determined to be 33.77%.[4] Therefore, by choosing a different material whose Shockley-Queisser limit is closer to this theoretical maximum of 33.77% we can still find some room for improvement.
High-efficiency solar cells are already used in areas where the energy output per unit of mass is more important than the production costs, such as in satellites and other spacecraft. Gallium Arsenide holds the world record for highest efficiency single-junction solar cell with a whopping 29.1% and is therefore the material of choice for such applications.[5]
A second way to increase the efficiency of solar cells is to combine multiple single-junction solar cells of a different material into one multi-junction solar cell.[6] Each individual junction absorbs a different part of the solar spectrum, and is still bound by their respective Shockley-Queisser limit. However, the combination of these single-junctions is not.[7] A promising candidate for a tandem (=two-junction) solar cell is the combination of Silicon with a perovskite material. Where perovskite refers to the crystal structure of this group of materials. Usually, such a material is a combination of an organic and an inorganic material, where the latter is often Lead or Tin joined with a halide (the halides are the elements in the second rightmost column of the periodic table: Fluorine, Chlorine, Bromine, Iodine and Astatine). Such a tandem solar cell has already reached efficiencies of 29.1% in practice.[8] Furthermore, the overall world record for solar cell efficiency was set at 47.1% in 2019 by a solar cell consisting of six different junctions.[9]
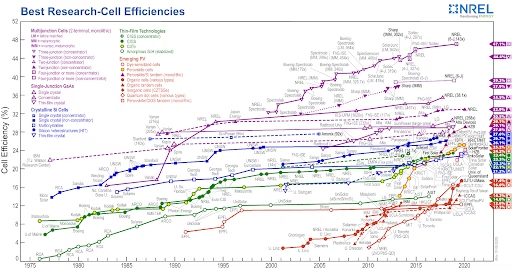
As we have seen, the answer to the question ‘can we make higher efficiency solar panels?’ can be answered with a resounding yes! However, this is not the right question to ask, because producing a solar panel only makes sense if it will produce more electricity in its lifetime than was consumed in its production process. Silicon based solar panels have the huge advantage that they are a) relatively easy to produce, and that b) Silicon is a very common element on earth, and it is relatively easy to extract. As a general rule of thumb, the more complex (e.g. more junctions) you make the solar cell, the more electricity it will cost to produce it. And at some point the efficiency gained by the increased complexity is no longer worth it.
Up to now we’ve been getting away with improving the existing technology of Silicon solar cells, however as we have seen this route is reaching a dead-end. To continue improving solar cells we have to use more exotic materials, which of course greatly increase the amount of electricity consumed in the production of the solar cell. The right question therefore should be ‘can we make viable higher efficiency solar panels?’, the answer to which regrettably is no at the moment.
Therefore, it is to be expected that the trend of increasing efficiency that we have seen in commercially available solar panels in the past, will stall and remain stalled for the foreseeable future. At least until some brilliant new breakthrough will greatly reduce the amount of electricity consumed in the production of multi-junction solar cells. All too often I see people pointing at this past trend of increasing efficiency, mistakenly expecting this trend to continue, and then concluding that some super-high efficiency solar panel will come to our rescue and aid us in the energy transition. It is good to hope, but we cannot base policy on such hope. We should stay realistic, and therefore assume that we will have to make do with the current Silicon solar cell technology in the energy transition.
How far can these solar panels get us? A study conducted in 2019 estimates that about 25% of the energy consumption in the European Union can be generated using rooftop solar panels alone![10] Considering that in that same year only 4% of the electricity produced in the EU was solar, there is still a lot of room for more solar energy.[11] However, as we shall see a theoretical possibility does not necessarily equate to a realistic, practical or timely solution, nor does the problem stop at generating the electricity, we also need to store and transport it.
The wind in the sails of the energy transition?
At first glance, one might think that the workings of a wind turbine are relatively easy. However, the problem very quickly becomes a lot more complicated once we aim to maximize the efficiency, and start to consider the effect that one wind turbine has on a neighbouring one in a wind farm. There are an incredible number of factors that affect the efficiency of a wind turbine, such as the shape and size of the blades, the strength but also stability of the wind, the losses incurred in the transmission mechanism and the generator, and also the shape and amount of obstacles in the vicinity, such as other turbines.[12].
The theoretical maximal attainable efficiency of any wind turbine is given Betz’s law. This law is based on the principle of conservation of mass and momentum, obviously the total amount of air-mass entering the turbine area should equal the amount of air-mass exciting this area on the opposite side. An equally obvious statement is that a turbine can never extract 100% of the energy contained in the wind, if it would the turbine would cause the wind to stop and air would accumulate just behind the turbine. This of course is completely unphysical, for the turbine to work it must be supplied with new air blowing in from the front, and therefore the air at the back of the turbine must make way. As a consequence there will always be some energy left in the wind after it exits the turbine. Betz’s law therefore concludes that no more than 59.3% of the energy contained in the wind can be extracted. Of course reality is not perfect, and factors such as friction cause energy to be lost. In practice a modern wind turbine has a peak-efficiency of around 44.5% to 47.4%.[13]
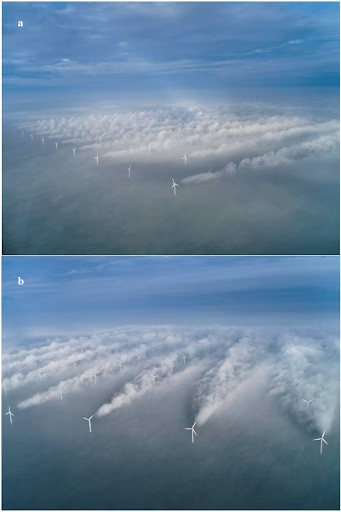
Wind energy realises its true potential at sea, where the wind is stronger, more constant, and more stable. Under such conditions a wind turbine can be up to 16% more efficient![14] Additionally, wind turbines do make some noise (about 45dB at a distance of 300 meters, which is slightly louder than a refrigerator)[15] this, together with the flickering shadow they produce, can become an annoyance to residents living very close to it. Such concerns are nullified at sea.
Combining multiple wind turbines into one big wind farm is advantageous in terms of energy transport. Since this would require one big cable instead of multiple smaller ones, especially for offshore turbines this obviously makes things a lot easier. The disadvantage of placing wind turbines close to one another is that the turbines will be, to some extent, in each other’s ‘wind shadow’, because once the wind has passed through one turbine it will naturally be weaker and more turbulent when it hits a second turbine. This turbulence can reduce the efficiency of wind turbines in the middle of a wind farm by as much as 20% compared to the turbines at the edges of the wind farm.[16] On the 12th of February 2008 rare weather conditions in the North Sea near Denmark caused this ‘wind shadow’ phenomena to be visualized by the mist forming in the wake of the wind turbines of the Horns Rev 1 wind farm, as can be seen in Figure 2.[17]
In 2009 a report from the European Environmental Agency claimed that there was enough usable wind, land and sea to meet the energy demand of the entire European Union ten times over.[18] While this study does not seem to take into account the effect that neighbouring wind farms have on each other, an effect which will most certainly become relevant when implementing wind energy on this massive scale,[19] this does show us the enormous potential that wind energy has, especially considering that in 2017 only 11.6% of the electricity used in the European Union had been generated using wind energy.[20]
Storage and the importance of a stable, robust, flexible and diverse electricity grid
“What to do when the sun doesn’t shine and the wind doesn’t blow” is the classic objection raised against solar and wind energy, especially the climate-change-denying right is very fond of using this argument to depict plans for combating climate change as unrealistic. However, it would be foolish to just discard this very real concern, merely because it was raised by reactionaries and the defenders of the vested interests of the fossil industries. At first glance the solution to this predicament seems obvious, simply store the generated energy when more is generated than is used for use on a rainy day. Sadly this is more easily said than done.
Electricity, or more accurately: electrical energy, itself cannot be directly stored, it has to be converted to some other form of (potential) energy which can be stored. This can for example be done using batteries (chemical energy), flywheels (mechanical energy), pumped-(water)-storage (gravitational energy), or by using electricity to create some kind of fuel such as Hydrogen (from water) or Methane (from water and carbon dioxide).[21]
Each storage technology has its own distinct advantages and disadvantages. However, they all have one thing in common: losses. Once again the party is ruined by the usual suspects: thermodynamics, friction and resistance. Any conversion of energy from one form to another will always cause energy to be lost, and any form of electricity storage will have at least two such conversions (from electricity, and back to electricity). Which means that storing electricity will inherently cause some of it to be lost, it is therefore always better to use it for something useful directly instead. Exactly how much electricity is lost depends on the storage technology that is used, it can be as little as 5% or as much as 60%.[22]
How much storage would we need? Of course, the more variable energy sources (=energy sources depending on factors outside of human control such as the weather), the more storage capacity would be required to compensate for the downtime of those sources. And in turn, the more storage capacity there is, the more additional energy sources required to actually fill this storage and compensate for the losses induced by the storage. A 2018 study shows us that, depending on the ratio of solar to wind energy, about 15% to 140% storage capacity is required to fully transition to only wind and solar energy.[23] Compared to wind energy, solar energy requires significantly more short term energy storage to keep the grid stable. And as such the 140% represents the scenario where the energy supply is almost entirely solar, and the 15% represents the opposite scenario where the energy supply is almost entirely wind. That being said, this study emphasizes the large uncertainty in such predictions due to their dependency on the flexibility and (local) transport capacity of the energy grid.
Diversity, flexibility, sustainability and robustness.
Can we provide the entire European Union with electricity using only solar, wind, and the therefore required storage capacity? As we have seen, yes, in theory we can! However, this is only part of the question. Can we reform the entire grid in this way in time to prevent total climate catastrophe? Can we mine and process all the required resources for the enormous amount of solar panels, wind turbines and storage? Does this strategy result in the most stable, most robust, most flexible energy grid? Is this strategy the most sustainable, or will it just cause another different crisis? In short, just because we can, should we disregard everything else and throw all our efforts into only solar and wind? Will this lead to the desired result of a stable, robust, sustainable energy grid?
The answers to these questions are often left unanswered. Authors often show that we can, and presume that this is enough and also means that we should. A 2018 report by the Dutch Ministry of Infrastructure and Water Management highlights the difficulties in meeting the exploding demand in rare-earth elements, and concludes that to avoid scarcity we must either reduce the consumption of these elements, redesign existing technologies to allow for way more recycling, or open new (European) mines.[24] And I haven’t even started about the abhorrent conditions in, for example, Congolese Cobalt mines, which are still the major supplier of Cobalt, an essential component in batteries.
Almost all countries are way behind schedule in the energy transition if they are to meet the goals agreed upon in the Paris accord.[25] One might therefore start to wonder if the mainstream approach focussing almost exclusively on solar and wind is way too narrow, and if we could not speed things up by choosing a strategy that allows for more different types of energy sources to be developed, built and implemented in parallel. Obviously if we were to broaden our approach we would also broaden the types of required resources, and therefore require less of those specific resources that we would otherwise require massive amounts of. This prevents bottlenecks in certain areas (such as the impending scarcity described in the previous paragraph) from slowing down the entire energy transition too much. To conclude, a broader approach to the energy transition equals a faster energy transition.
Additionally, an electricity grid that relies only on solar and wind is necessarily way more decentralized compared to our current grid consisting of relatively few centralized energy sources. Some authors present this as an advantage, after all this reduces our dependence on large electricity corporations. I say they are wrong, for starters because their argument is moot, the dependency on large electricity corporations is not removed, it is merely shifted towards large manufacturers and distributors of solar panels and wind turbines. Secondly, this creates a culture of ‘every man for his own electricity’, which is not only morally wrong from a Marxist perspective, it is also a bad idea from a technological perspective. Because an electricity grid is a complex thing where the amount of electricity sent into the grid must always be actively kept in balance with the amount of it that is used, if the balance is off too much a power outage is the result. This means that to prevent power outages, a constant flow of information is required between an enormous amount of sensors, switches, storage facilities and production facilities. This challenge would become exponentially more difficult the more decentralized and individualistic the electricity generation is.
An electricity grid is per definition a collective thing, completely decentralizing it would be as ill-advised as decentralizing the railways. It might work, but it would almost certainly be less stable and less robust than a centrally coordinated approach. In addition, it would be far from trivial to convert the existing electricity grid which has a more tree-like structure consisting of huge cables from the sources branching off into increasingly smaller cables, to the more web-like structure required for a more decentralized grid. Such a conversion, while technically possible, requires time, resources and effort, time which is running out and resources which might be better used elsewhere. Therefore, we would be better off opting for a strategy that requires less changes to the existing electricity grid, in other words a strategy that requires less decentralization.
Furthermore, let’s ask ourselves exactly why it is that we are in our current predicament. Obviously the answer is our current, almost exclusive, dependence on fossil fuels. The ‘exclusive’ part is very important here, in 2018 fossil fuels were still used for 70.2% of the electricity generation.[26] Imagine if our starting point had been an electricity grid that was only 50% dependent on fossil fuels where the remaining 50% was generated using something else. In this case it would of course be easier to remove the dependence on fossil fuels, than if 100% of the electricity would be generated using fossil fuels. The conclusion is that the larger the share of a certain energy source in the overall electricity generation, the harder it is to remove the dependence on it. What is the wise lesson to be learned here? It is that a diverse electricity grid is more flexible, and that therefore to avoid problems like this from becoming this huge in the future we should aim to create an electricity grid consisting of an as diverse as possible collection of energy sources.
An electricity grid based solely on solar and wind would in this aspect suffer from the exact same problem. If for whatever reason we would need to dial back the use of wind or solar we are once again in trouble. Or imagine what would happen if a massive volcano eruption covered all solar panels in an area spanning thousands of kilometres with a small layer of ash, reducing their efficiency by as much as 50%.[27] What if a huge storm (which is happening more frequently due to climate change) causes a large number of wind farms to temporarily be out of service. The critic will say that when the solar is out of service, the wind will take over, and when the wind is unavailable, the solar will take over. I would answer that this presumes that the other source is able to take over, which is not the case unless you either build a significant overcapacity of solar and wind, or create yet even more storage. As if we weren’t having enough trouble producing enough solar panels and wind turbines. I would propose an alternative solution: diversify! The more diverse the electricity grid is, the better it is able to cope with any one source being temporarily unavailable. Hence a diverse electricity grid is more robust.
An introduction to nuclear energy
A 2013 study concluded that nuclear energy has over the years prevented the emission of 64 gigatonnes of carbon-dioxide-equivalent (all greenhouse gasses taken together and expressed in terms of carbon dioxide), and in doing so has prevented 1.84 million air pollution-related deaths. In this number the authors have already subtracted the direct and indirect deaths from all nuclear disasters.[28]
Furthermore, a 2014 study by the Intergovernmental Panel on Climate Change (IPCC) compared the total carbon-dioxide-equivalent released per kWh in the construction and during the life-time of different energy sources. A very relevant comparison because even during the construction of energy sources that are otherwise emission-free, fuel and electricity are used. The results of the study are shown in Figure 3 where we can see that nuclear energy scores are comparable to wind energy with a median of about 12 grams of carbon-dioxide-equivalent per kWh. Solar energy (PV=photovoltaics) on the other hand scores four times worse with about 41 to 48 grams of carbon-dioxide-equivalent per kWh. As a side note, the same figure also shows us that biomass is not a solution, scoring only marginally better than coal. And that Carbon Capture (CCS) is also not a viable method to combat climate change still having a very large carbon-dioxide-equivalent footprint.[29]
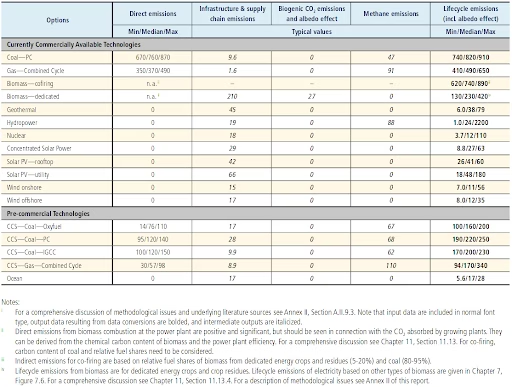
Dogmatically focussing exclusively on solar panels and wind turbines would be incredibly short-sighted. We would exchange an absolute dependency on fossil fuels with an absolute dependency on the weather, and would be required to completely redesign the existing electricity grid. We should instead focus on a more sustainable, faster and long-term approach, where we aim to create an electricity grid which is as diverse as possible. Don’t get me wrong, I’m very fond of solar and wind energy, but we cannot pretend that simply putting solar panels on our roofs, and wind turbines on our shores will solve our problem, as we have seen the problem is more complex and a strategy focussing exclusively on solar and wind would require massive investments in storage technologies and changes to the existing electricity grid. Nor should we presume that such a strategy would be fast enough to prevent climate catastrophe, or result in the best possible electricity grid. Therefore, I argue that we should diversify our approach, in order to speed up the energy transition, and to create a more stable, robust, and flexible electricity grid as a result. So in addition to solar and wind energy, we should also develop and deploy other (less known) non-fossil energy sources, such as: hydroelectricity,[30] geothermal energy,[31] tidal power,[32] wave power,[33] and yes nuclear energy.[34] And as we shall see in parts 2 and 3, the latter is nothing to be scared of.
2: Historic context, and the development of nuclear fission energy
To understand the current state of nuclear energy, we must first travel back to its origins at the beginning of the previous century. The historic context of the parallel development of both nuclear fission and fusion energy with weapons of mass destruction, lead to some design choices, development priorities, and an understandable culture stigma, which to this day still have consequences for the current state and perception of nuclear energy.
Frédéric Joliot-Curie
In the early 1930s Ernst Rutherford and his colleagues discovered that splitting Lithium atoms released an enormous amount of energy.[29] It did not take long for physicists to realise that if this were to be done with an element that released more energy than had to be put in to get it to split (elements heavier than Iron), that a chain reaction could ensue releasing enormous amounts of energy. Such chain reactions were experimentally confirmed by Frédéric Joliot-Curie in Paris when he showed that Uranium bombarded with neutrons would split and cause more neutrons to be emitted than were absorbed. The observant reader might recognize part of the last name of Joliot-Curie, they would be correct, this is indeed the son in law of the pioneers of radioactivity Marie and Pierre Curie.[35]
The year of this discovery was 1939, a year that is more commonly infamous for the start of the second world war. Some physicists at the time were all too aware of the potential evil uses of their discoveries. Leo Szilard, a Hungarian physicst, who migrated to the US to escape the Nazi terror, urged Joliot-Curie not to publish his findings lest the Nazis get their hands on it.[36] Where other physicists, such as Enrico Fermi, followed Szilard’s advice and self-censored their research, Joliot-Curie continued the Curie tradition of publishing all their work openly for the benefit of the entire scientific community and published in April 1939.[note]
Despite his decision to publish anyway, Joliot-Curie, an outspoken communist, did not underestimate the risks of his discovery. Earlier that year, in January 1939 he wrote to fellow physicist, Abram Ioffe, in the Soviet Union, alerting him that the Nazis had been performing successful experiments with nuclear fission.[35] And on the 30th of October, Frédéric and his wife Irène Joliot-Curie (also a nuclear chemist/physicist and a communist) took the decision to stop publishing their work after all and placed all their documentation on nuclear fission in the vaults of the French Academy of Sciences, where it remained until 1949.[37]
After the Nazi-invasion of France the Joliot-Curies smuggled their working documents and materials to England, Irène sadly contracted tuberculosis and spent most of the war recovering in Switzerland, Frédéric stayed behind and joined the French resistance. During the Paris uprising in August 1944 he made Molotov cocktails for his fellow rebels, the main weapon against tanks. And after the liberation the Joliot-Curies were finally able to reunite again.[35]
Because he was a communist, Frédéric got purged from most of his positions after the war. And like many comrades with them, the Joliot-Curies’ contributions to science, society and the resistance movement went mostly unappreciated in the west.[29] This is why I have chosen to go slightly off topic here and give the Joliot-Curies their due credit.
Nuclear weapons and the Blitzkrieg
The 1st of September 1939, the day that the Nazis invaded Poland, did not only mark the start of the second world war, it was also the day the second Uranverein (Uranium Association) was formed. This shows us that Szilard’s worries about a possible Nazi nuclear weapon were not at all unjustified. Luckily the Nazis actually never had serious ambitions to create a nuclear weapon. Werner Heisenberg was one of the members of the Uranverein, he writes that physicists at the second meeting said that: “in principle atomic bombs could be made.... it would take years.... not before five." Heisenberg himself continues:
I didn't report it to the Führer until two weeks later and very casually because I did not want the Führer to get so interested that he would order great efforts immediately to make the atomic bomb. Speer felt it was better that the whole thing should be dropped and the Führer also reacted that way.[38]
The Nazis trusted in a swift victory, and as such the lengthy development process of a nuclear weapon was mostly considered a waste of resources. By the time it was done, they would have won already, or so they thought. Furthermore, the Nazis were convinced that the Americans were also not even close to having a working nuclear weapon.[29] Additionally, even if the Nazis did have serious ambitions to create a working nuclear weapon, they were already behind in this race because in April 1933, shortly after Hitler gained power, the ‘Law for the Restoration of the Professional Civil Service’ was passed, banning ‘non-Aryans’ and political opponents from being civil servants.[39] The direct effect was that many of the people affected by this law fled the country, among them many scientists who took their knowledge and expertises with them.
Not only that, science in Germany, and physics in particular, had been suffering from nationalist tendencies for some time. This surfaced in the distinction between Deutsche Physik (German Physics) and Jüdische Physik (Jewish Physics), where the latter was considered inferior and bad science. The works of prominent physicists such as Albert Einstein and Niels Bohr were censored and ridiculed because of their authors Jewish descent. Even the ‘Aryan’ scientists who stayed behind in German occupied territories were affected by this. Heisenberg, for example, was repeatedly harassed for teaching Einstein’s theory of relativity and quantum mechanics, both of which were considered ‘Jewish physics’. Himmler eventually forbade attacks against Heisenberg, realizing that Germany could not afford to lose him as well, after Heisenberg’s mother had phoned Himmler’s mother to ask her if she would please tell the SS to give “Werner” a break.[40]
Science does not thrive under censorship and oppression, the Nazis proved as much. It is highly likely that even if the Nazis had ambitions to create a nuclear weapon, that such attempts were doomed to fail. Because, as it turns out, it is rather difficult to do any nuclear research at all if you consider quantum mechanics and relativity to be inferior sciences. Despite this, the Nazis did perform some experiments in the field of nuclear physics, for applications in submarines. And after the invasion of Norway the Nazis had access to heavy-water factories there.[38] Heavy-water is water where one of the Hydrogen atoms is the heavier isotope of Hydrogen: Deuterium. And it is a useful neutron moderator, which means that it can reduce the speed of neutrons in a nuclear reactor without capturing them; these slower neutrons are more likely to propagate the nuclear chain reaction. Furthermore, the Nazis also had access to the Uranium mines in the Czech Republic, and had banned the commercial sale of the Uranium mined there. In hindsight we might be able to conclude that a Nazi nuclear weapon was very unlikely, Szilard and others however could not know this and had more than enough reason to worry.
The stain of the Manhattan project
Szilard’s worries drove him to take action. On the 12th of July 1939 he and his, also Hungarian, colleague Eugene Wigner visited Einstein, and convinced him to sign a letter to the Belgian Ambassador to the US. The then Belgian Congo was at the time a major source of Uranium ore, and therefore a potential target for the Nazi Afrikakorps (Africa Corps), and Einstein happened to know the Belgian royal family personally.[41]
A second letter was addressed to president Franklin Roosevelt, it is this letter which will become famous as the Einstein–Szilard letter. In this letter Einstein and Szilard express their concerns about a possible Nazi weapon of mass destruction:
In the course of the last four months it has been made probable – through the work of Joliot in France as well as Fermi and Szilárd in America – that it may become possible to set up a nuclear chain reaction in a large mass of uranium, by which vast amounts of power and large quantities of new radium-like elements would be generated. Now it appears almost certain that this could be achieved in the immediate future.
This new phenomenon would also lead to the construction of bombs, and it is conceivable – though much less certain – that extremely powerful bombs of a new type may thus be constructed. A single bomb of this type, carried by boat and exploded in a port, might very well destroy the whole port together with some of the surrounding territory. However, such bombs might very well prove to be too heavy for transportation by air.[42]
As it turns out Einstein and Szilard would be proven wrong about that last statement when a breakthrough in the UK massively reduced the required mass to sustain a nuclear chain reaction (the critical mass, to use the technical term).[41]
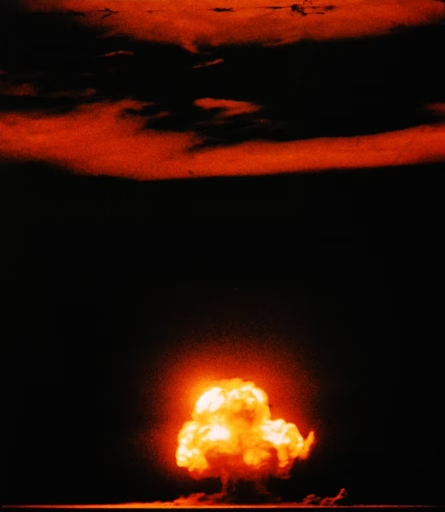
An immediate result of the letter was the creation of the Advisory Committee on Uranium, and a huge investment in the experiments of Szilard and Fermi. Later theoretical successes in the UK (the MAUD reports) eventually prompted the authorisation of a full scale development plan for a nuclear weapon in January 1942. In June of that same year the US military took over the project, and rebranded it to the infamous Manhattan project.[41]
And so it came to be that on the 2nd of December 1942 in the middle of Chicago the first nuclear reactor became operational. Not with the goal to provide humankind with massive amounts of electricity, but with the explicit aim to kill and destroy. An ugly stain which has been besmirching the entire field of nuclear physics ever since.
Not long after, in 1943, the first industrial Plutonium production reactor became operational on the Colorado river near Hanford. It was the Plutonium produced here that would be used in the first atomic bomb that exploded in the desert of New Mexico. And it was this Plutonium that on the 9th of August 1945, in the historical climax, razed Nagasaki to the ground.[43] [44] The cold war was now inevitable, and the fate of the reputation of nuclear physics was sealed.
The cold war
A: I think [the bombing of Hiroshima and Nagasaki] made it very difficult for us to take the position after the war that we wanted to get rid of atomic bombs because it would be immoral to use them against the civilian population. We lost the moral argument with which, right after the war, we might have perhaps gotten rid of the bomb.
Let me say only this much to the moral issue involved: Suppose Germany had developed two bombs before we had any bombs. And suppose Germany had dropped one bomb, say, on Rochester and the other on Buffalo, and then having run out of bombs she would have lost the war. Can anyone doubt that we would then have defined the dropping of atomic bombs on cities as a war crime, and that we would have sentenced the Germans who were guilty of this crime to death at Nuremberg and hanged them?
But, again, don't misunderstand me. The only conclusion we can draw is that governments acting in a crisis are guided by questions of expediency, and moral considerations are given very little weight, and that America is no different from any other nation in this respect.
So Szilard tells us in an interview with U.S. News & World Report titled ‘President Truman Did Not Understand’[45] (a very interesting read by the way). Though the bombing of Japan might have ended one war, they also immediately signalled the start of the next one. Having observed the destructive power of the new weapons in the US arsenal, the USSR immediately accelerated their own nuclear projects, with a nuclear arms race as direct consequence.
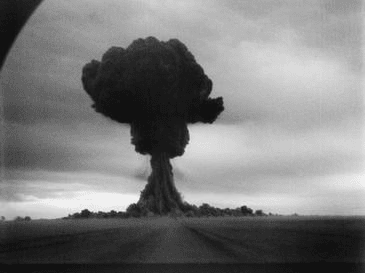
As early as April 1942 the USSR had already started working on a nuclear weapon, because Russian physicist Georgy Flyorov had noticed that the Germans, British and Americans had stopped publishing papers on nuclear physics. However, due to the war with the Nazis in their own territories, the project was not very high on the priorities list and was therefore not progressing very rapidly. This would immediately change following the bombing of Japan, the Soviet Politburo took direct and complete control of the project, and just over a year later, on the 29th of August 1946, the Soviets also performed their first nuclear weapon test in what is now Kazakhstan.[29]
And so an intense arms race was started, where the destructive capacity of nuclear weapons kept increasing and increasing. For every single technological and scientific question, the path chosen was the path that would lead to best applicability in weapons.[46] The questions physicists on both sides had to ask themselves was “how do we destroy as much as possible”, instead of “how do we provide as many people as possible with as much electricity as possible efficiently and safely”. The remnants of the decisions taken in this historic context can still be seen in the state of nuclear reactors today. In part 3 we will analyse some of the paths taken, and also some of the paths not taken, and what this means for the current state of nuclear energy. Furthermore, in part 4 we will trace the origins of some of the worst nuclear accidents back to this desire to destroy.
3: The current state and potential of nuclear energy
Q: Would most other nations, including Russia, have done the same thing we did, confronted with the same opportunity to use the bomb?A: Look, answering this question would be pure speculation. I can say this, however: By and large, governments are guided by considerations of expediency rather than by moral considerations. And this, I think, is a universal law of how governments act.
Prior to the war I had the illusion that up to a point the American Government was different. This illusion was gone after Hiroshima.
Perhaps you remember that in 1939 President Roosevelt warned the belligerents against using bombs against the inhabited cities, and this I thought was perfectly fitting and natural.
Then, during the war, without any explanation, we began to use incendiary bombs against the cities of Japan. This was disturbing to me and it was disturbing many of my friends.
Q: Was that the end of the illusion?A: Yes, this was the end of the illusion. But, you see, there was still a difference between using incendiary bombs and using the new force of nature for purposes of destruction. There was still a further step taken here - atomic energy was something new.
I thought it would be very bad to set a precedent for using atomic energy for purposes of destruction. And I think that having done so we have greatly affected post war history.[45]
Szilard makes an interesting point here about governments, which would make a fascinating topic for a different article, but let me say this much about it here: I sure hope he is wrong about this “universal law”, I would hope that a hypothetical future workers government in a war with a fascist nation would make a different choice, and not resort to such drastic measures as wiping whole cities of the face of the earth.
Asides from that, Szilard’s prediction about the precedent that was set by the atomic bombings and the nuclear arms race are spot on. To this day, one cannot talk about nuclear energy without someone bringing up nuclear weapons, the two are intrinsically connected in the minds of many people. However, to what extent is this link really fundamental from the point of view of physics? We will explore this question further in this third part.
Let’s start with the working principle behind nuclear energy, it is in fact relatively simple. One takes a relatively heavy atom, shoots a neutron into it causing it to become unstable and split into two (or more) parts. This process releases energy because the resulting products combined have a lower energy than the original starting atom (this is true for all product elements heavier than Iron). The energy is released in the form of heat, some neutrons, photons (gamma radiation), electrons (beta radiation) and Helium ions (alpha radiation). The released neutrons will collide with other atoms, causing those to split as well, and thus even more heat to be produced. And so a nuclear chain reaction is created. The resulting massive amounts of heat can be used to drive a turbine and generate electricity.[34]
A couple of things are important. First of all, for every neutron adsorbed at least one neutron should be released again, otherwise the chain reaction will die out. On the other hand, too many neutrons will cause the amount of reactions to increase exponentially, which becomes a problem when the amount of heat generated becomes a lot bigger than the amount of heat that can be removed. If the reactor’s fuel is in a solid state, this can eventually cause the fuel to melt, a meltdown![47]
Not only the amount of neutrons, but also the speed of these neutrons is important. Some fuels are more susceptible to collide with slow neutrons, others are more stable (i.o.w. have a larger energy barrier) and only spit upon collision with faster neutrons. To control this speed so called neutron moderators are used, which slow down the neutrons, ideally without absorbing too many of them.
Therefore, the art of controlling a nuclear reactor, is the art of controlling the amount and speed of the neutrons in the reactor. Some reactors are designed such that physics will cause the amount of neutrons to automatically decrease when the fuel gets too hot, such reactors are called inherently safe.
Thorium, Uranium and Plutonium
This brings us to a first design choice: the fuel. As a general rule of thumb, all odd isotopes of the actinides are directly usable as a fuel in a nuclear fission reactor. The actinides are the elements in the bottom row of the periodic table of elements, and are the heaviest elements. An isotope is a version of an element with a different number of neutrons in the nucleus. As an example, Actinium, the first actinide, consists of 89 protons, and either has 136, 137 or 138 neutrons. These isotopes would then be called Actinium-225, Actinium-226, and Actinium-227, where the number behind the element name is the sum of the protons and neutrons (89+136=225, 89+137=226 and 89+138=227).[48]
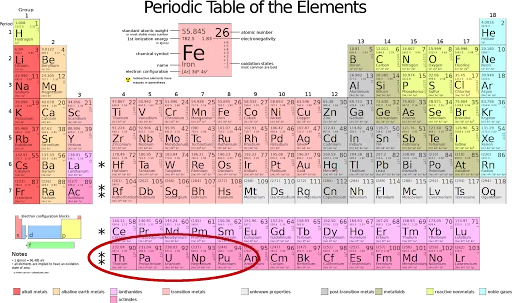
Of these actinides only two can be found on earth in relevant amounts: Thorium and Uranium. Uranium is found in a mix consisting mostly of 99.27% Uranium-238 and 0.72% Uranium-235,[49] the slightly lighter Thorium on the other hand consists of 99.98% Thorium-232[50] and is 4 times as common on earth.[51] Of these three isotopes only Uranium-235 has an odd number of neutrons and is directly able to sustain a nuclear chain reaction by itself, we therefore call it fissile. The other two mentioned isotopes, Thorium-232 and Uranium-238, are not directly fissile. However, they are fertile, meaning that they can be converted into a fissile isotope by capturing neutrons (Uranium-233 and Plutonium-239 respectively, both formed after capturing one neutron and emitting two electrons, i.o.w. double beta decay).[52]
Some reactors or other applications require a larger percentage of Uranium-235 in the fuel than can be found in natural Uranium ore. Mostly these are designs where too many neutrons are absorbed by things that are not fissionable, such as the moderator or cooling fluid. In such cases it is necessary to increase the amount of neutrons in the reactor to ensure that every fission will still cause at least one other fission. One way to do this is to increase the concentration of the fissile part of the fuel, or in other words, in the case of a Uranium fuel, to increase the concentration of Uranium-235 compared to Uranium-238, this is what is called enrichment. How much enrichment is required depends on the application. For bombs you’ll want as much explosive power in as little as mass as possible, which means that you’ll want the concentration of Uranium-235 to be as large as possible, in other words you’ll want weapons-grade Uranium (more than 85% Uranium-235). On the other hand, if the aim is to create Plutonium you’ll want the bare minimum of enrichment, since it is the Uranium-238 that will produce the Plutonium-239 upon neutron capture. For electricity production only low amounts of enrichment are required (less than 20%), and some electricity reactor designs don’t need any enrichment of the fuel at all and can use natural Uranium (CANDU and other pressurized heavy-water reactors), such reactors have other ways to ensure that there are enough neutrons in the reactor (such as the use of heavy water as neutron moderator).[53]
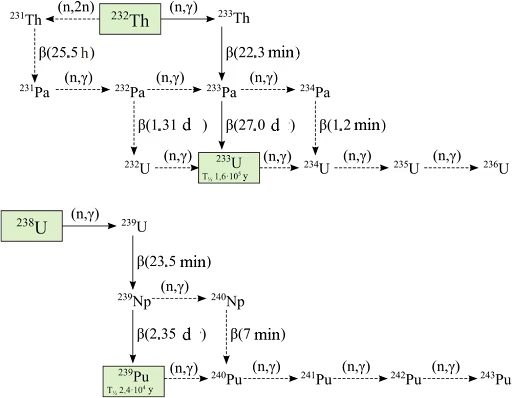
That being said, Uranium-235 is not the only possible fuel. Thorium-232 , was already shortly mentioned above, unlike Uranium-235, it will not fission immediately upon capturing a neutron, instead it will absorb this neutron and become Thorium-233. This Thorium-233 is very unstable and will very quickly decay to Protactinium-233 by emitting an electron (beta radiation). The Protactinium-233 will then decay in the same way again to form Uranium-233, which is fissile and will therefore split and release energy upon capturing another neutron.[54] Note the difference between decay and fission, the two are often confused. Radioactive decay is the general term for any unstable isotope releasing any kind of radiation to become more stable. Fission is a type of radioactive decay, which sometimes occurs spontaneously in heavy unstable isotopes, but can also be induced by neutron capture as we have seen above. So all fission is radioactive decay, but not all radioactive decay is fission, other forms of radioactive decay are; the emission of a highly energetic photon (gamma radiation), the emission (or capture) of an electron (beta radiation), or the emission of a Helium-4 ion (alpha radiation). The fission products that are created by the splitting of an unstable isotope, are of course significantly lighter but are often still unstable isotopes and therefore will often still undergo some form of (alpha, beta, gamma) radioactive decay before eventually becoming a stable isotope.
A similar process to what we have seen for Thorium-232 occurs in Uranium-238, which becomes Uranium-239 after capturing a neutron. This decays to Neptunium-239, and again to Plutonium-239, which is fissile.[55] Uranium fuelled nuclear reactors will always contain some Uranium-238 (how much depends on the amount of enrichment), and therefore such reactors will always produce some Plutonium-239 as a byproduct. This is not a problem because Plutonium-239 is also fissile and is therefore a fuel for the nuclear reaction, and as such can just be ‘burned up’ as well. Plutonium, however, has another more sinister application, which is where it gets its bad reputation from.
Plutonium-239: The isotope of choice to kill and destroy
All three of the active fuel isotopes mentioned above are in principle also usable to create a nuclear weapon; Uranium-235, Uranium-233 (from Thorium-232) and Plutonium-239 (from Uranium-238). However, in practice the element of choice for this application is almost always Plutonium-239.[56] This is because the minimal mass (critical mass) required to sustain a chain reaction in Plutonium-239 is 5 times smaller than that of Uranium-235.[57] This means that a Plutonium based weapon can be significantly lighter than a Uranium based one. Additionally, it is a lot easier to separate Plutonium from Uranium than it is to enrich natural Uranium to the high concentrations of Uranium-235 required for weapons-grade Uranium.[56]
The third isotope, Uranium-233, is comparable to Plutonium-239 in terms of critical mass, but requires a purity that is 1300 times higher to make a functional weapon.[58] This is because the nuclear reaction that produces Uranium-233 also produces a bit of Uranium-232 as a byproduct. This Uranium-232 produces highly energetic gamma radiation which interferes with electronics and therefore significantly limits the applicability of Uranium-233 in weapons.[54] A similar problem occurs in the production of Plutonium-239, where there will always be a bit Plutonium-240 produced as a byproduct. This Plutonium-240 is problematic as well because it tends to spontaneously fission, producing neutrons which could make the bomb go off prematurely if the concentration of Plutonium-240 is too high.[55] However, the amount of Plutonium-240 that is acceptable in a Plutonium-239 based weapon is 1300 times higher than the amount of Uranium-232 in a hypothetical Uranium-233 weapon.
So if you’d want to make nuclear weapons you’d choose Plutonium-239 as the active isotope, and if you can’t get your hands on that, you’d revert to highly enriched Uranium. It is thus no coincidence that the first large scale nuclear reactors that were built both in the US and the USSR were Plutonium production reactors.[59] These reactors never produced any electricity, they couldn’t because they were not designed to do this and therefore lacked the necessary infrastructure to convert heat to electricity. Furthermore, to some extent the goals of creating Plutonium-239 or electricity are opposites. Because to create Plutonium-239 the fuel should not stay too long in the reactor, otherwise the Plutonium-239 would be ‘burned up’ or too much Plutonium-240 would be created.[56] If such a reactor would be optimized for the production of electricity instead, the fuel would be replaced way less often, because in this case it would in fact be beneficial for the Plutonium-239 and Plutonium-240 to fission as well, and therefore create additional heat and electricity. In fact, the longer you can keep the fuel in the reactor the more of the heavy actinides produced will fission again, which means more heat and thus electricity produced, and also that less of these heavy actinides will end up as nuclear waste (the heavier actinides are usually problematic in the waste because of their long life times).
After the first Plutonium production reactor opened in 1943 (Hanford, US) it took 11 years before the first electricity producing reactor became operational (1954, Obninsk, USSR).[59] The difference in timespan with how long it took to build the first Plutonium production reactors, once again shows us how skewed the priorities were. Furthermore, almost all of the early reactors that did produce electricity, only had this as a secondary goal, where the primary aim was to create Plutonium. Historically speaking nuclear energy is clearly a byproduct of nuclear weapons, however it would be a mistake to assume that this implies that this link is fundamental and also true from the viewpoint of physics. The political and historical context caused certain choices to be made and priorities to be set, but choices and priorities they definitely were and nothing more than that. It would in fact have been possible to completely develop nuclear energy without ever making a single bomb.
When one aims to make a bomb, one optimizes the reactor and the surrounding infrastructure (e.g. enrichment and other separation facilities) for this purpose and tries to create as much Plutonium-239 as possible, and of course this has had its effect on which designs and ideas received the most funding and were eventually implemented, the consequences of which can still be seen today. In the next section we will explore one of the many alternate paths not taken: Thorium.[60]
Thor’s Hammer: an unused power that never got its due attention
Weinberg realized that you could use thorium in an entirely new kind of reactor, one that would have zero risk of meltdown. ... his team built a working reactor .... and he spent the rest of his 18-year tenure trying to make thorium the heart of the nation’s atomic power effort. He failed. Uranium reactors had already been established, and Hyman Rickover, de facto head of the US nuclear program, wanted the plutonium from uranium-powered nuclear plants to make bombs. Increasingly shunted aside, Weinberg was finally forced out in 1973.
Science writer Richard Martin writes this about physicist Alvin Weinberg.[29] Weinberg filed the patent on a conceptual light water reactor together with Eugene Wigner,[34] to this day the most common type of nuclear reactor.[59] Light water, as opposed to heavy water, is just regular water and is used in this type of reactor as a neutron moderator and as a coolant. The use of light water requires that the Uranium fuel is slightly enriched, since light water absorbs more neutrons than heavy water.
Weinberg questioned the wisdom of the continued upscaling of his own design, because he understood all too well the critical weakness in his design. If something were to go wrong, such as a critical failure in the water pumps, and the cooling would become inadequate, then the fuel rods could overheat and melt: A Meltdown! For the original application this design was made for, the navy, this wasn’t really a problem because it is easy to guarantee the availability of water for a boat or submarine, since it is quite literally swimming in its own cooling fluid. However, Weinberg was not a big fan of applying his design on land.[34] Expressing his concerns cost him his job as director of the Oak Ridge National Laboratory, Weinberg himself writes:
[Congressman] Chet Holifield [head of the Joint Committee on Atomic Energy (JCAE), which oversees the US Atomic Energy Commission (AEC)] was clearly exasperated with me, and he finally blurted out, "Alvin, if you are concerned about the safety of reactors, then I think it may be time for you to leave nuclear energy." I was speechless. But it was apparent to me that my style, my attitude, and my perception of the future were no longer in tune with the powers within the AEC.[60]
For applications on land Weinberg proposed a different kind of reactor, one which did not use any water, one using Thorium as the fuel instead of Uranium, one that was inherently safe. This design was based on earlier designs made for the air force, and though a nuclear propelled aircraft never became a reality the design itself was tested successfully on land. The test reactor at the Oak Ridge National Laboratory used Uranium-233 (made from Thorium) dissolved in a Fluoride salt as the fuel, and operated successfully from 1965 to 1969, and in doing so, demonstrated the feasibility of the concept.[61]
Such a liquid Fluoride Thorium reactor has several advantages. First of all, the lack of water automatically removes the risk of contaminated water from the reactor entering the ecosystem.[62] Secondly, and most importantly, there is 0 risk of a meltdown. For one because the fuel is already in a dissolved liquid state in normal operation. Furthermore, such a reactor includes a plug made from that same Fluoride salt which will melt when the Fluoride-fuel solution in the reactor gets too hot. The melting of this plug will automatically drain the reactor and store the solution safely in large container tanks. No human or electronic action is required, just gravity. Once the solution is outside of the reactor it can expand, which will automatically cause it to cool down. To start the reactor up again, all one has to do is pump the solution back into the reactor.[63]
Additionally, such a reactor produces almost no Plutonium, or other heavy actinides for that matter. Because for this to happen Uranium-233 would have to capture 6 consecutive neutrons and become Uranium-239 so it can decay to Plutonium-239. The odds that an Uranium-233 atom does this are very low, since for every neutron capture there is a chance that the isotope will fission instead. And the capture-to-fission ratio of Uranium-233 is smaller than that of Uranium-235 and Plutonium-239.[58] Moreover, due to its liquid nature, the heavy actinides that it does produce can just stay behind in the reactor and be burned up, only the fission products have to be extracted as waste.
Furthermore, the radioactivity of the waste that a liquid Fluoride Thorium reactor produces returns to background levels way faster than that of a conventional light water reactor. Because, as we saw earlier, it produces less heavy actinides, which are the most problematic isotopes in nuclear waste. 83% of the waste from a liquid Fluoride Thorium reactor has a half-life of a few hours to days, the remaining 17% only has to be stored for 300 years compared to the ten thousands of years required for the waste from more conventional reactors. Compared to the Uranium fuel cycle, the Thorium fuel cycle has a radiotoxicity that is 10.000 times smaller.[63]
Moreover, the liquid Fluoride Thorium reactor operates at low pressures, and the pressure does not increase a lot even if the reactor runs several hundreds of degrees too hot. Thorium is four times as abundant as Uranium. And the liquid nature of the reactor allows for refuelling and separation of the waste fission products without having to turn the reactor off.[29]
Sadly, Thorium never truly got its chance to shine. The technology for Uranium reactors was already established, and Thorium could not satisfy the military’s demand for Plutonium. Thus, in 1973, the US pulled the plug on the research into Thorium.[60]
Nuclear fusion energy: the energy source of the future?
Nuclear fission energy is popularly abbreviated to nuclear energy. However, nuclear energy is wider than just nuclear fission energy, it encompasses all energy generation involving nuclear reactions, and as such also includes nuclear fusion energy. No article on nuclear physics could be complete without at least talking a bit about nuclear fusion, so let’s dive into that here.
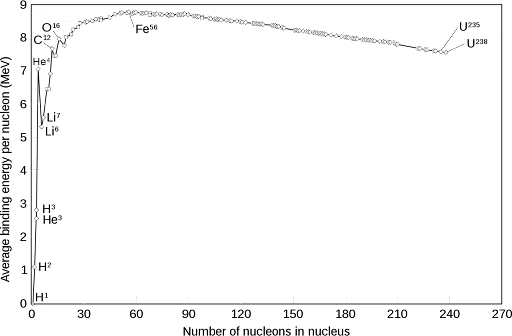
As we have seen, very heavy nuclei release energy when they split, the opposite is true for the very light nuclei that gain energy when they fuse together. The middle between these two extrema is at Iron-56, which has the highest binding energy, indicating that of all the nuclei this is the most stable one and would take the most energy to completely take apart. Figure 8 shows this binding energy as a function of the size of the nuclei.[29]
It is exactly such a nuclear fusion process that takes place in the sun (and other stars). Four Hydrogen-1 nuclei fuse together to eventually form one Helium-4 nucleus. The process releases radiation and an enormous amount of heat which keeps us warm and provides us with light on earth. In theory this process could be replicated on earth to create heat and thus electricity. However, the technical challenge is the enormous pressure and the temperature of millions of degrees Celsius required to accomplish this.
Once again an explosive history
Some authors condemn nuclear fission energy because of nuclear weapons and waste, only to praise nuclear fusion energy in the next paragraph as the alternative that is free of all those things that make nuclear fission nasty. This is unbelievably and painfully ironic, first of all because nuclear fission actually does produce some radioactive waste. And secondly because many nuclear weapons actually are based on nuclear fusion, the so called thermonuclear weapons or hydrogen bombs.
The fusion reaction produces quite a lot of radiation, and this radiation is absorbed by the structure of the reactor itself, upon absorbing this radiation some of the nuclei in the structure will become different nuclei which may be unstable and radioactive. The end result is that, first of all the structural integrity of the reactor degrades over time, and secondly that at the end of the lifetime of the reactor some of the structure will remain radioactive for about 100 years. Granted, this is a relatively short time period, but the claim that nuclear fusion produces no radioactive nuclear waste is plain false.[64]
The history of nuclear fusion starts in 1933, when Mark Oliphant was able to create Helium-3 using a proton-accelerator. And as if the corruption of nuclear fission was not enough this breakthrough would soon also lead to new even more destructible weapons. The first Soviet atomic bomb, in 1949, came way sooner than the Americans expected, and so the Americans quickly started designing a new type of bomb that would top the existing fission based bombs. The idea was simple, take an existing Uranium-235 bomb and add some Deuterium and Tritium (Hydrogen-2 and Hydrogen-3 respectively). After the first stage, the Uranium stage, would explode, the temperatures would quickly become high enough for the Deuterium and Tritium to fuse, releasing more energy and more neutrons, the extra released neutrons would in turn cause even more fission in the Uranium, releasing even more energy.[65]
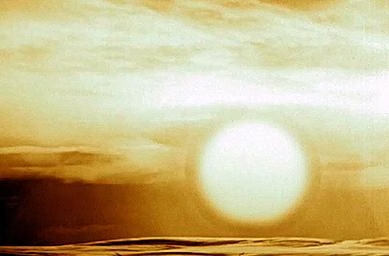
On the 25th of May 1951, a first proof-of-concept bomb successfully exploded on an island in the middle of the pacific ocean. It proved to be twice as destructive, compared to the same bomb without the fusion phase. Shortly afterwards, on the 1st of November 1952 the first true thermonuclear bomb exploded, which was 450 times more destructive than the bomb that was dropped 7 years earlier on Nagasaki.[65] However, this explosion pales in comparison to the bomb that the USSR brought to explode over Nova Zembla on the 30th of October 1961. The code named Tsar bomb was 5 times as powerful as the before mentioned American thermonuclear bomb, and resulted in a fireball of more than 8 kilometres wide. The resulting mushroom cloud was 67 kilometres high, 7 times higher than Mount Everest. The heat from the explosion was still noticeable at distances of 270 kilometres, windows shattered as far as Finland and Norway.[66] To this day, this was the most powerful man-made explosion, an eyewitness describes:
The clouds beneath the aircraft and in the distance were lit up by the powerful flash. The sea of light spread under the hatch and even clouds began to glow and became transparent. At that moment, our aircraft emerged from between two cloud layers and down below in the gap a huge bright orange ball was emerging. The ball was powerful and arrogant like Jupiter. Slowly and silently it crept upwards... Having broken through the thick layer of clouds it kept growing. It seemed to suck the whole Earth into it. The spectacle was fantastic, unreal, supernatural.[67]
The future?
Just as with nuclear fission, the military research into nuclear fusion has spawned a parallel research into applications for the production of electricity. And, just as with nuclear fission, there are a lot of different reactor designs both with their own advantages and disadvantages, a full comparative analysis of which would be outside of the scope of this article. The interested reader can find more information in the sources list.[64]
What all designs have in common is that a huge amount of energy is required to get the fusion reaction to start. And none of the reactors which have been built so far have come close to breaking even. The world record was set by the Joint European Torus near Oxford; this reactor operated for about one second in 1997, consumed 700 megawatts of electricity, to produce 16 megawatts of heat. Despite that this was not even near breaking even it was still a major accomplishment. And in 2007 the research was continued by the start of the International Thermonuclear Experimental Reactor project in southern France. The expectation is that when this reactor is finished, it will be able to operate for about 1 second in 2035, consuming 300 megawatts of electricity to produce 500 megawatts of heat.[68]
Perhaps in the far future nuclear fusion will be able to contribute to the electricity generation, however we cannot expect this to happen any time soon. Nuclear fusion is still very much in the experimental phase, and with the promise of only 1 second of operation in 2035, we cannot expect nuclear fusion to be able to contribute to the energy transition at all. Additionally, it is very difficult to find funding for such long-term projects under capitalism where short-term profits are the norm, and as such promises such as X megawatts of electricity in 20YZ should always be taken with a grain of salt because such promises are made in the context of trying to lure in funding within a system that expects short-term results. Personally, I would be very surprised if nuclear fusion would be able to contribute to the electricity generation in any significant amount within my lifetime.
Part 4: Reactor designs, the brilliant and the stupid
In these early days [of nuclear energy] we explored all sorts of power reactors, comparing the advantages and disadvantages of each type. The number of possibilities was enormous, since there are many possibilities for each component of a reactor—fuel, coolant, moderator. The fissile material may be 233U[ranium], 235U[ranium], or 239P[l]u[tonium]; the coolant may be: water, heavy water, gas, or liquid metal; the moderator may be: water, heavy water, beryllium, graphite—or, in a fast- neutron reactor, no moderator. I have calculated that, if one counted all the combinations of fuel, coolant, and moderator, one could identify about a thousand distinct reactors. Thus, at the very beginning of nuclear power, we had to choose which possibilities to pursue, which to ignore.
In this quote from Weinberg’s book The First Nuclear Era: The Life and Times of a Technological Fixer he explains that nuclear energy is not a monolithic thing, it is instead a whole field of research.[69] It is of course to be expected that the historic context has had an enormous effect on, as Weinberg says, which possibilities were pursued and which were ignored. Some authors who oppose nuclear energy treat nuclear physics as if it were exclusively the science of pressurized light water reactors (the most common type of nuclear reactor). They would proceed to describe, in their eyes, the disadvantages of ‘nuclear energy’ when in fact they are describing only pressurized light water reactors. In doing so, these authors prove that they have not done a single bit of research, since a simple scroll through Wikipedia would have revealed that this point of view is wrong and that there are in fact dozens of types of nuclear reactors, each with several subtypes and each with their own distinct advantages and disadvantages.[70] Such authors dogmatically oppose anything that even contains the word ‘nuclear’, and will yell buzzwords like ‘nuclear weapons’, ‘radioactive waste’ and ‘Chernobyl’ at you if you dare defend any kind of nuclear reactor.
We have already seen that the link between nuclear weapons and nuclear energy is purely historical, and not fundamental, technological or physical. It was the political choices and historic context which has pushed technology towards optimization for the creation of nuclear weapons, and not some fundamental law of physics. Some designs for nuclear reactors have already been described: the (pressurized) light water reactor, the (pressurized) heavy water reactor, and the liquid fluoride thorium reactor. A complete comparative analysis of all types of nuclear reactors would be outside of the scope of this article (the interested reader can find more reading material in the sources list), the take away point is that nuclear energy is not a monolithic thing, and should not be treated as such by authors who wish to engage in a serious scientific discussion. The liquid fluoride thorium reactor was highlighted because it shows that Uranium is not the only possible fuel, and because it is my personal favourite. However, it is not the only modern (generation IV) design, nor is it the only inherently safe design.[71]
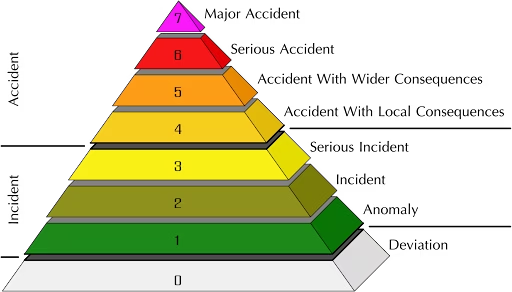
Since nuclear accidents are always part of the usual critique against nuclear energy, we will analyse the major nuclear accidents in this fourth part, using the knowledge obtained in the first three parts. The International Atomic Energy Agency (IAEA) has defined the International Nuclear Event Scale (INES) to categorize nuclear accidents. It is a scale from 0 (Deviation) to 7 (Major Accident), throughout history there has been one level 6 (Serious Accident) event, and two level 7 events. In my opinion the scale is somewhat vague (and according to Wikipedia I am not the only one that thinks the INES is confusing), but let’s use it here anyway.[29]
Kyshtym: haste makes waste
When the USSR started to seriously invest in nuclear weapons, after the bombing of Hiroshima and Nagasaki, they were already behind in this race. The Soviet physicists had almost no experience with nuclear weapons, and were under large pressure to catch up with the Americans. In the period from 1945 to 1948, the Mayak Production Association was constructed with extreme haste and with very little concern for other factors such as safety or environmental impact. The aim was to create as much Plutonium as quickly as possible, all other concerns were secondary.
This light water reactor used the water from lake Kyzyltash directly for cooling, only to dump the contaminated water directly back into the lake. Additionally, the nearby lake Karachay was used as a dumping ground for the waste products. It did not take long for this area to become the most contaminated place on earth.
And as if that wasn’t enough, on the 29th of September 1957 the cooling of one of the storage tanks failed and was not repaired. The temperature steadily rose, eventually resulting in an explosion and a radioactive cloud contaminating areas hundreds of kilometres away. Because the Plutonium production reactor was considered a state secret, the evacuation of 10.000 people only took place one week later and proceeded without any explanation. It would take another 18 years before the details became publicly known.
The IAEA categories this as a level 6 accident. Though it is unclear how many people died as a result of the explosion and the prolonged contamination of the area, estimates are of the order of hundreds. The entire area was declared to be a nature reserve, and with that excuse the Soviet regime forbade anyone from entering.[72]
The root cause of the accident can obviously be found in the haste and pressure to produce Plutonium and catch up with the Americans in the arms race. This caused carelessness and the cutting of all the corners that could possibly be cut, which is of course bound to go wrong at some point. They simply did not care about a possible accident, or about the environmental damage they caused, the eyes were only on the prize of winning the arms race.
Chernobyl: a disaster that started on the drawing board
The worst nuclear accident in history is that of Chernobyl, categorized as a level 7 by the IAEA. This disaster shows like no other that some reactor designs are just a very bad idea. The Chernobyl power plant consisted of four high-power channel-type reactors, a design that, first of all, utilizes an exceptional amount of water. Every channel requires about one and a half bathtubs of water per minute, reactor number 4 had 1661 of such channels.
Additionally, the control rods, which are supposed to reduce the amount of neutrons in the reactor by capturing them, were made out of graphite at the bottom and neutron absorbing boron carbide at the top. Graphite is a neutron moderator meaning that it slows neutrons down which increases the chance of the neutron causing a Uranium-235 to fission, therefore the graphite part does the opposite of what the control rods are supposed to do, it increases the reactivity of the core instead of decreasing it. The graphite bottom was added to prevent neutron absorbing water from filling the space left vacant when the control rod was in the extracted position. So the idea was that in the down position the boron carbide part of the control rod would be in the core, absorbing the neutrons and therefore slowing down, and eventually stopping, the reaction. And in the up position the graphite part of the control rod would be in the reactor, moderating the neutrons and increasing the reactivity of the core. A good idea in principle, it does however have an unintended side effect: If the control rod is completely extracted (graphite and boron carbide part) then the space left vacant will fill up with water anyway. Which means that when the control rod is inserted back in again, it is the neutron moderating graphite part that will first displace the neutron absorbing water before the neutron absorbing boron carbide part enters the reactor. This means that putting the control rods back in from the completely extracted position will first increase the reactivity of the core before decreasing it, a strange and unexpected property for a control rod to have.
Furthermore, the reactor had a dangerously high positive void coefficient. This coefficient indicates how the reactor responds to bubbles of boiling cooling fluid. A positive void coefficient indicates that the reactivity increases when there is a void (bubble) where the cooling fluid is supposed to be, a negative void coefficient on the other hand indicates that a void decreases the reactivity of the core. So a negative void coefficient is good, because when the reactor becomes too hot and therefore the amount of bubbles increases, the reactivity will automatically decrease. The high-power channel-type reactors had a very positive void coefficient, which means that when the reactor starts to overheat, more bubbles will form, causing more fission reactions to occur, causing more heat, causing more bubbles, etc. In other words, a positive feedback loopback, something you don’t really want in a nuclear reactor.[73]
One might wonder, if the design has these critical flaws, why build it anyway? Well first of all, the design was an adaptation of the existing military Plutonium production reactors, so the technique was semi-familiar. It was adapted in such a way that it could perform a hybrid function and produce both Plutonium and electricity. As we have seen before these goals don’t really align well, if you want to create electricity you want continued uninterrupted operation, and you want to burn up as much as possible, including the Plutonium, you therefore keep refuelling to a minimum. On the other hand, if you want to create Plutonium for weapons you’ll need to refuel often to prevent the Plutonium-239 from already fissioning in the reactor, and to prevent too much Plutonium-240 from building up. So how was this contradiction resolved? Well the reactor was designed such that it could be refuelled without turning it off. And that was one of the major selling points of the high-power channel-type reactors, it allowed for individual channels to be replaced while the reactor was operational. Furthermore, the use of the neutron moderator graphite, which absorbs very little neutrons, allowed for the use of very low enriched Uranium as fuel.[74]
For this design a guaranteed supply of cooling water was essential. Therefore, Chernobyl had several back-up diesel generators to supply the water pumps with electricity in the case of an emergency situation. However, it would take 60 to 75 seconds for the generators to start up and take over the electricity generation for the water pumps. That does not sound like a lot, but an inadequate cooling of about a minute is more than enough for things to become problematic for such a reactor with a strongly positive void coefficient that is in normal operation already notoriously difficult to handle. To resolve the situation, it was hoped that the main steam turbines could be used to bridge the gap. Since it would take some time for the turbine to spin down when the reactor switched off, it might just be able to produce enough electricity to power the water pumps while the diesel generators were starting up. This of course needed testing, and after three failed tests a fourth test was planned for the 25th of April 1986.
Due to unexpected downtime of a different power plant, the test was postponed by 11 hours, which meant that now the night shift had to perform the test instead of the day shift that was prepared for it. As the test procedure described, the reactor was slowly powered down. However, at some point the reactor power suddenly dropped completely, why this happened is unknown. To restore the power to the level required to complete the test most of the control rods were manually raised. As part of the plan extra water pumps were turned on, which increased the water flow, but also the inlet temperature of the water since the water now spent less time releasing its heat in the cooling towers. Therefore, the safety margin was reduced, and the increased water flow caused the temperature in the reactor to drop which reduced the amount of bubbles and thus decreased the power output of the reactor again. To restore the power level once again, two circulation pumps were turned off, and even more control rods were extracted. The result was an extremely unstable reactor, nearly all of the 211 control rods had been extracted manually, including all but 18 of the fail-safe manually operated rods of the minimum 28 that were supposed to remain fully inserted.
Despite this, the test was continued anyway. The steam supply to the turbines was cut off, causing the turbines to slowly spin down, and the diesel generators started to turn on and take over. As the power supply to the water pumps dropped, so did the water flow, increasing the temperature in the reactor, causing more bubbles, causing the temperature to rise even more. The idea was that 39 seconds into the tests the diesel generators would be able to supply enough of the water pumps with electricity to sustain a sufficient water flow.[73]
However, 36 seconds into the test an emergency full shutdown was manually initiated, who did this and why exactly is unclear because those involved died soon after. Such an emergency stop is called a SCRAM in English, which is either derived from the verb, or refers back to that the first nuclear reactor (Chicago Pile-1) actually had an emergency control rod tied to a rope hanging above the reactor and a man with an axe standing next to it to cut the rope in case of an emergency: “Safety Control Rod Axe Man.”[75] The Russian name (AZ-5) is way less interesting, and translates to “Emergency Protection of the 5th Category”. In any case, a SCRAM immediately causes all control rods to be completely inserted into the reactor automatically, with the intent of completely stopping the nuclear reaction as fast as possible. However, as we recall, the control rods of the high-power channel-type reactors have a rather special feature which causes the reactivity of the reactor to first increase before it decreases.
The graphite part of the control rods got inserted into the reactor, replacing the neutron absorbing water, and increasing the reactivity of the reactor. This contra-intuitive feature of the reactor had been observed before in this reactor design, but had never been communicated with the personnel of the Chernobyl power plant (in fact, earlier partial meltdowns and other (nuclear) accidents in Chernobyl unit 1 and the Leningrad power plant, which was of the same design, were treated as state secrets and never communicated with the workers at the plant).[74] Combined with the unstable state the reactor already was in, this proved to be the final straw. The reactor overheated, some of the fuel rods fractured, blocking the further insertion of some of the control rods. The reactor output rose to 10 times its nominal output. The steam pressure built up and caused an explosion, more channels ruptured, the coolant supply lines got severed, and the fuel got released into the coolant. A second more powerful explosion occurred two to three seconds later, ejecting most of the reactor core, the exposed hot graphite caught fire when it came into contact with oxygen.[73] Engineer Alexander Yuvchenko was in his office when disaster struck, and survived, in a fascinating interview he describes his experience:
To get a clearer idea of what had happened we walked outside. What we saw was terrifying. Everything that could be destroyed had been. The entire water coolant system was gone. The right-hand side of the reactor hall had been completely destroyed, and on the left the pipes were just hanging. That was when I realised that Khodemchuk was definitely dead. The place where I was told he'd been standing was in ruins. The huge turbines were still standing, but everything around them was rubble. He must have been buried under that. From where I stood I could see a huge beam of projected light flooding up into infinity from the reactor. It was like a laser light, caused by the ionisation of the air. It was light-bluish, and it was very beautiful. I watched it for several seconds. If I'd stood there for just a few minutes I would probably have died on the spot because of gamma rays and neutrons and everything else that was spewing out. But Tregub yanked me around the corner to get me out of the way. He was older and more experienced.[76]
It took 36 hours before the evacuation of the nearby Pripyat began. Residents were told this was temporary, but soon after, the evacuation of the area became permanent. To this day 2600 km2 of Ukraine is uninhabited due to the large amount of radioactivity in the area.[77] In the first three months after the disaster 31 people died of acute radiation syndrome (=radiation sickness). Furthermore, it is estimated that from the five million inhabitants of the contaminated area, about 4000 died as a result of radiation induced cancer.[73] (The exact number is heavily debated, some estimate it to be lower, some estimate it to be way higher. In this article we will stick to the IAEA number of 4000, which was reaffirmed in 2008, based on 20 years of research and on the actual increase of cancer cases. We will not indulge in any extravagantly ridiculous numbers pushed by opponents of nuclear energy based on models which are not supported by peer-reviewed science, models which assume that even the tiniest amount of radiation will increase the risk of developing cancer.)
Fukushima: how a natural disaster became a nuclear disaster
The nuclear reactors at Fukushima were of the boiling light water type, meaning that in this reactor the water boils in the reactor and the resulting steam drives a turbine. In contrast the water in a pressurized light water reactor does not boil, instead the water stays in liquid form, and exchanges its heat with a secondary water circuit which is not pressurized and does boil, the resulting steam of this secondary circuit drives a turbine.[78] Compared to Chernobyl’s high-power channel-type reactors, the reactors at Fukushima did not use graphite, instead the light water was used both as the coolant and as the neutron moderator.[79]
On the 11th of March 2011 a massive earthquake occurred near Japan’s largest island. The resulting forces applied to the Fukushima reactor were well above the design limits for continued operation, and therefore reactors 1,2 and 3 switched off automatically, reactors 4, 5, and 6 had already been powered down for routine inspection and refueling. As a result of the earthquake one of the two connections to the electricity grid of reactors 1,2 and 3 broke, therefore 13 emergency diesel generators kicked in to supply the cooling of the reactors with enough power.
So far nothing serious has happened. However, about 50 minutes after the earthquake a huge tsunami with waves of up to 14 meters splashed over the 10 meter high seawall (the 35 meter high natural seawall had been removed to ease construction). This caused a flooding of the basement, which for some reason was where the reactors’ emergency diesel generators were (engineers had actually pointed out way earlier that the location of the generators left them vulnerable to flooding). The switching station that provided a connection with three different backup generators located higher up the hill also flooded and was no longer functional. The cooling power was now entirely dependent on a set of batteries, which quickly ran out. Additional portable generators and batteries came late due to poor road conditions as a result of the earthquake and the tsunami, and when they did arrive the flooding made it impossible to connect them to the cooling pumps. Eventually the cooling pumps stopped and reactors 1,2 and 3 began to overheat (even when such a reactor is off it still generates some heat due to radioactive decay occurring in the fuel rods). This caused a meltdown in reactors 1,2 and 3.
Furthermore, the high temperatures in the reactors caused the Zirconium coating of the fuel rods to react with steam to form Hydrogen. The concentration of Hydrogen kept increasing and eventually caused an explosion in reactor 1 on the 12th of March, a similar explosion occurred in reactor 3 on the 14th and in reactor 4 on the 15th because reactor 4 happened to be connected to reactor 3 via a ventilation shaft. Reactor 2 did not explode.
Despite being a level 7 event on the INES scale nobody actually died of radiation sickness directly, though many people were injured by the explosions or received serious radiation burns. Moreover, tens of people did die as a result of the evacuation, mostly the elderly and patients from local hospitals. Additionally, large amounts of radioactive material were released into the environment, and in 2015 the tap water in Tokyo was still significantly more radioactive compared to other Japanese cities. TEPCO, the company owning the power plant, had been warned several times both internally and externally about the risk of flooding due to a tsunami. On the 12th of October 2012 TEPCO admitted that they had been negligent, and that they had not taken the necessary precautions out of fear that doing so would trigger lawsuits and protests. They also acknowledged that they could have reduced the impact of the disaster, or even prevented it entirely, if they had adhered to international norms and recommendations. Several other similar nuclear power plants were able to achieve a cold shutdown, despite that some of those had also been flooded by the tsunami, the Onagawa Nuclear Power Plant was closer to the epicentre of the earthquake but survived just fine thanks to its 14 meter high sea wall.[80]
In the same interview we saw earlier, when asked about what he thinks of nuclear power, Yuvchenko hits the nail on the head when he answers: “I'm fine about it, as long as safety is put head and shoulders above any other concern, financial or whatever. If you keep safety as your number one priority at all stages of planning and running a plant, it should be OK.”[76] We see time and time again that when things go wrong we can trace the cause to that safety was not the primary concern. In Kyshtym it was the thirst for Plutonium that led to safety and environmental concerns to be completely cast aside. In Chernobyl the desire to create both Plutonium and electricity led to an unstable and unsafe hybrid design, whose oddities and flaws were never communicated with the workers at the plant because of a hierarchical and bureaucratic culture of secrecy. In Fukushima it was financial and reputational concerns that led to inadequate precautionary measures being taken.
We have already seen that the history of nuclear physics has been riddled with ‘other concerns’ besides safety and the prosperity of humankind, the consequences of which have given the entire field a bad reputation. We have also seen that there are many different reactor designs, some safer than others, some even inherently safe. The conclusion is that there is no reason at all to declare that all of nuclear energy is ‘inherently dangerous and unsafe’, as some opponents of nuclear energy tend to do. To end this section as we began, with a quote from Weinberg:
We nuclear people have made a Faustian bargain with society. On the one hand we offer—in the catalytic nuclear burner (i.e., the breeder [reactor])—an inexhaustible source of energy. Even in the short range, when we use ordinary reactors, we offer energy that is cheaper than energy from fossil fuel. Moreover, this source of energy when properly handled is almost non polluting. Whereas fossil-fuel burners emit oxides of carbon, nitrogen, and sulfur... there is no intrinsic reason why nuclear systems must emit any pollutant except heat and traces of radioactivity. But the price that we demand of society for this magical source is both a vigilance from and longevity of our social institutions that we are quite unaccustomed to.[69]
But…. What about the waste?
There will always be a bit of waste, because every fission of a fissile element results in waste products which usually are unstable and will therefore be radioactive to some extent before they eventually decay to a stable isotope. How much waste depends on the design of the reactor, we have already seen that the liquid fluoride thorium reactor produces less waste compared to the more conventional light water reactors, and the radioactivity of the waste that it produces goes down to background levels way faster.
Spent nuclear fuel will contain the products of the fission reaction, and some actinides consisting of the remaining fuel and some heavier actinides that were formed due to neutron capture of the fuel elements. The exact composition of the spent fuel depends on the original fuel, but also on the amount and speed of the neutrons in the reactor during operation. It is mostly those heavier actinides which are problematic because of their long life times and high amounts of radioactivity.[81] Luckily, those heavier actinides can in principle be ‘burned up’ by forcing them to fission, creating lighter more manageable isotopes.
The reprocessing of nuclear fuel separates the light fission products from the remaining fuel and other heavy elements, the reprocessed fuel can then be used as fuel once more. This recycling increases the amount of energy that can be extracted from the fuel by as much as 60 times, and also has the benefit of removing the problematic heavy actinides from the waste that eventually needs storing.[82] Using this method 96% of spent fuel can be recycled, the remaining 4% are the fission products which should be stored until their radioactivity decreases to background levels.[83]
How long this waste should be stored depends on how well it was reprocessed and on the original composition of the spent fuel before reprocessing (and thus on the used fuel, and on the type of reactor). The easiest way to store this waste is to simply bury it in a dry earthquake-free place. In 2015 Finland has started the construction of such a deep geological repository, the aim is that it will be open for about 100 years during which it can be filled with nuclear waste. After this period the tunnel will be sealed, providing a long-term storage for the waste 520 meters under the ground.[84] Problem solved!
Luckily the energy density of nuclear energy is extremely high, and therefore the amount of waste is very low. A typical 1000 megawatt nuclear power plant produces merely 27 tonnes of unprocessed spent fuel in one year.[83] That is the equivalent of 20 m3, and can be reduced to as little as 3 m3 by reprocessing. That is a miniscule amount for a whole gigawatt over a whole year.
“To not saddle future generations with nuclear waste” is an argument one often hears against nuclear energy. In the above we have seen that this argument is complete rubbish, first of all because the amount of radioactive waste is not a lot, and secondly because good long-term solutions exist. Contrary to carbon dioxide and other gaseous waste, which is a problem for which there still is no good solution at all.
Part 5: Stigmas, populism, opportunism, and capitalism
In the first part we have seen that the strategy of focussing solely on solar and wind energy, is too slow. We have also discussed the advantages of a more diverse electricity grid. And concluded that to effectively combat climate change as fast as possible and create a robust electricity grid in the process, we should diversify our approach.
In the second part we have discussed the history of nuclear physics, to conclude that the historic context during the early days of nuclear physics has had a significant effect on the development direction of the field. We continued this line of thinking in the third part, where we discussed the physics of nuclear energy and nuclear weapons, to conclude that the historic context of the past still has an effect on the technological, scientific, and societal status of nuclear energy.
In the fourth part we analysed the major nuclear accidents, and showed that their causes can be traced back to priorities other than the safety being at the top of the list. Putting this in the historic context and scientific background of parts two and three respectively, we concluded that the occurrence of these disasters does not imply that nuclear energy is inherently dangerous or unsafe. Additionally, we saw examples of reactor designs which in fact are inherently safe, shortly discussed the futuristic potential of nuclear fusion energy, and talked about what to do with the radioactive waste.
In the original Dutch version, this fifth part contained an analysis of the positions of the Dutch left political parties on the matter of nuclear energy. And showed that almost all of these parties were presenting misleading statements, or in some extreme cases outright lies. Since this is not really relevant for the international reader, this part has been omitted. Instead this fifth part now contains an examination of the points brought forward by comrade Eddie Ford in two of his articles titled “Swords into ploughshares?” and “Expensive, dangerous, unnecessary” in which he opposes nuclear energy.[85] [86] Conveniently, comrade Ford’s arguments are not that different from those one finds in the party programs of the Dutch left, or from any other item opposing nuclear energy for that matter. Comrade Emil Jacobs has also written a reply to the first article titled “Luddite delusions” which contains some very good points and I highly recommend reading it.[87] The section on stigmas and the interests of the established capital is left mostly unaltered.
Stigmas and the interests of the established capital
The stigma
If nuclear energy is so amazing, then why are we not massively using it now? Part of the answer to this question can be found in history and in particular in the cold war. The huge amount of weapons of mass destruction constructed, formed a real existential threat for practically all of humankind. And though the acuteness of a potential nuclear mutual annihilation has faded, the threat is still there. In 2019 there still were 13865 operational nuclear weapons worldwide, of which 3750 were deployed with operational forces, 90% of those weapons were owned by the US and Russia.[88]
Rightly so, this led and continues to lead to massive protests. Socialists and communists should obviously oppose weapons of mass destruction. However, such protests often target nuclear (fission, and occasionally fusion) energy as well, which is unjustified. They cancel everything that involves techniques and science that even remotely touches with the fabrication of nuclear weapons, even though there is no fundamental or physical reason that nuclear energy must lead to the creation of nuclear weapons.
If there are no nuclear reactors of any kind or enrichment facilities, there are no Plutonium production reactors, and so no Plutonium or weapons-grade Uranium for nuclear weapons. Though not technically incorrect, such reasoning cuts all corners and ignores all details to conclude that the baby should be thrown out with the bathwater. In fact one can apply such reasoning to draw any number of absurd conclusions: I could write a whole article describing the horrors of drone strikes in the middle east, and I could compile a list of all the cities that were completely destroyed by aerial bombings since the invention of the air plane. I could continue describing terrible aviation accidents and as the cherry on the cake describe how on the 11th of September 2001 even civilian aircraft were used as weapons. To eventually conclude that all of aviation is inherently unsafe, and that we should not spend a single penny on research into this field since this can only lead to more deadly weapons, more death, and more destruction. I will leave it as an exercise to the reader to think about how we can use similar absurd reasoning to conclude that we should stop buying matches, starting from the destruction of Dresden in the second world war.
For every new technology, improvement or knowledge two things are always true. Firstly that mistakes will be made, and secondly that an imperialist power will try to find ways to abuse it. The former is a reason to be careful, take precautionary measures, and to think about what we are doing, and we can and should learn from the inevitable mistakes. The latter is a reason to be constantly vigilant, and we can and should oppose such abuse when it happens. What we should not do is reactionarily oppose such advances all together, else we will get stuck and can never make progress
And the capital that financed it
Capitalism commodifies everything, electricity is no exception. The physical unit Joule is reduced to a product either sold for consumption, or utilized for the production of other commodities. Which brings us to the remaining part of the answer to the question we asked ourselves in the previous section, the interests of the established capital: the fossil industries.
To this day the large majority of electricity is still produced using fossil fuels. The industry that supplies these fuels obviously has an interest in preserving this market. And as such, it has engaged in an active lobby against nuclear energy since the 50s. Let’s see an example: in 1954 during a meeting of the Rockefeller Foundation Board of Trustees, several of the trustees asked the president of the American National Academy of Sciences to start a research into the effects of radiation on public health.
Luckily for them they did not have to leave their seats to make this request because the president of the National Academy of Sciences happened to be a member of the Rockefeller Foundation Board of Trustees. The large majority of the assets of the Rockefeller Foundation are in stocks in the oil industries, and as such this ‘research’ of course concluded that “atomic radiation, no matter how small the dose, harms not only the person receiving it but also all his descendants”. Which is complete nonsense and scaremongering, because ionizing radiation is only harmful in large doses, something which was also known at the time. Additionally, these so-called ‘outstanding scientists’ had conveniently forgotten that background radiation exists, and that the amount of radiation a person is exposed to is never zero. The ‘findings’ of this study received wide coverage in the media. The New York Times went as far as to publish six different articles about the matter in their 13th of June 1956 edition. Coincidentally, at the time the publisher of the New York Times also happened to be a member of the Rockefeller Foundation Board of Trustees.[89]
And so the fossil industries laid the foundation of irrational extreme radiophobia. Some fossil fuel companies even went as far as to sponsor their opponents, the environmental organisations, if they had an explicit anti-nuclear stance.[90] And with great success, to this day the stigma against nuclear energy is still very much alive. To once again quote Weinberg:
My former colleague, William Clark, has likened the public’s frenzy over small environmental insults to the fear of witches in the later Middle Ages. Some million certified “witches” were executed because they could not prove that they had not caused harm to someone or something. In the same way, since one cannot prove that tiny amounts of radiation did not cause a particular leukaemia—for that matter one cannot prove that they caused it either—those who wish to succumb to low-level phobia succumb. As a result nuclear energy […is] under siege. Not until the low–level controversy is resolved can we expect nuclear energy to be fully accepted.[91]
The siege Weinberg describes is partly due to radiophobia and partly due to fear for the annihilation of humankind by nuclear weapons. These fears are financed by the established capital and they are fed by nuclear disasters caused by skewed priorities of the governments and companies that operate nuclear power plants. And it is here that we find the difficult task for socialists and communists, we must fight against weapons of mass destruction, and at the same time we must also expose the stigma sponsored by the established capital that is preventing a fast and effective energy transition away from fossil fuels.
Craziness and delusion?
Comrade Ford starts his article by describing the Fukushima and Kyshtym disasters, comrade Jacobs has already added an important footnote to some of the numbers thrown around there that are supposed to impress us. I happen to agree with comrade Ford’s assessment on the strange way nuclear accidents are rated by the INES scale, ranking Fukushima above Kyshtym and equal to Chernobyl seems a bit strange. However, we have already analysed those same disasters in part four, so let’s continue on.
The next section of Comrade Ford’s article is aptly titled ‘craziness’. He first raises the question of nuclear waste and makes a valiant effort to present the problem as unsolvable, when in fact we have already seen in part four that a perfectly fine solution does in fact exist, and that the amount of waste is extremely small for the amount of electricity gained. “Deep underground in repository sites? Inside mountains?” he ironically asks, to which I would like to unironically reply: Yes!
Next he raises the hypothetical scenario of a terrorist attack on a nuclear power plant. However, if we were to extrapolate this reasoning then we cannot do anything any more: Did we stop building skyscrapers after 9/11, did we stop flying? Did we stop building dams out of fear that terrorists would blow it up and flood downstream villages? Did the Netherlands stop building homes below sea level out of fear the terrorists would blow up the dykes and flood half the country? If we allow such reasoning to dictate our actions and prevent us from building nuclear reactors and therefore prevent us from stopping climate change, then the terrorists have won.
At the end of this section comrade Ford comes to the core of his argument:
Of course, in terms of history, the real reason for nuclear power was the development of nuclear weapons - the two cannot be disentangled. Indeed, it was an open secret that in 1956 Calder Hall was designed primarily for the production of weapons-grade plutonium. Furthermore, the idea was for Britain to become an ‘independent’ nuclear power not reliant on the US - which did not succeed. Making the actual bomb was the easy bit, but developing an effective delivery system was an entirely different matter.
And here he completely misses the mark. As we have seen in part two it was not that nuclear power was developed for the sole purpose of making nuclear weapons. In fact, nuclear weapons came way before any working nuclear power plant, proving that to make nuclear weapons it was never necessary to make a working nuclear power plant. It is true that most reactors constructed at the time, such as Calder Hall, performed a dual function to produce both Plutonium and electricity, such design choices however do not prove an intrinsic link between nuclear weapons and nuclear energy. Furthermore, we have seen in part three that such a link in fact only exists historically, not physically, and therefore comrade Ford’s claim that “the two cannot be disentangled” is false.
In his next section comrade Ford claims that it is “delusional” to think that “nuclear power is some sort of answer to global warming”, he states that such belief is ‘techno-utopian’. I would like to ask comrade Ford how we should combat climate change? If comrade Ford thinks that we can just put solar panels on our roofs and wind turbines on our shores and pretend the problem is solved then I would claim that he is in fact the techno-utopian. In the same paragraph comrade Ford claims that “nuclear power is also inherently dangerous”, it does not grace the comrade that he treats the entire field of nuclear energy as a monolithic entity. Additionally, we have already seen in parts three and four that this statement is rubbish, and I will not waste any more words on it here since comrade Ford did not bother to substantiate his claim with anything but the observation that accidents have happened.
I agree with comrade Ford’s assessment that a working nuclear fusion power plant is still a distant dream. However, I would like to point out the irony of first presenting the nuclear waste problem as unsolvable only to later “not want to categorically rule out nuclear fusion”, what is comrade Ford going to do with the radioactive waste from a hypothetical nuclear fusion power plant at the end of its life-time? And why would comrade Ford’s solution to this problem not be applicable to waste from a nuclear fission power plant? Or has the comrade simply not given that matter any thought? Furthermore, as we have seen in part three, nuclear fusion also has a direct historical connection to weapons of mass destruction, in fact the most destructive of weapons, the thermonuclear weapons, are based on nuclear fusion. How is it that comrade Ford dismisses nuclear fission because of its military history but does not apply the same reasoning consistently to nuclear fusion? Extrapolating his own flawed reasoning, he should oppose nuclear fusion as well.
In his last paragraph, comrade Ford proposes to power the UK using solar panels in the Sahara. Comrade Jacobs has already pointed out that resistance exists, and that therefore such a solution would be far from optimal. Physicists are working very hard to find a room-temperature superconductor at low pressures, however the large-scale application of superconductivity might be a dream that is even further away than a working nuclear fusion power plant.
Comrade Ford has one more argument up his sleeve that we skipped over before because I think that it is a truly ridiculous argument, the costs. It never ceases to amaze me that opponents of nuclear energy, no matter how far on the left, will all of a sudden care about whether something is economical or profitable under capitalism when the subject is nuclear energy. And to be honest I couldn’t care less if nuclear energy is economical or profitable to some capitalist, what I care about is combating climate change as fast and effectively as possible. But since comrade Ford has brought up the argument, let’s debunk it anyway. Wikipedia tells me that “it would cost between $6 billion to $9 billion for a 1,100 MW plant,”[34] lets humour comrade Ford and take the higher number of $9 billion, that would give us 8.2 dollars per Watt, a nuclear power plant lasts about 60 years before it needs a significant refurbishment so that would be 0.14 dollars per Watt per year. Now let’s direct our browser to Amazon and buy ourselves a solar panel, let’s humour comrade Ford again and buy a nice and cheap one, 100 dollars for a 100 Watt solar panel. And let’s assume that our really cheap and crappy solar panel lasts the full average lifetime of about 10 years, that gives us 0.10 dollars per Watt per year. And what do you know, more or less the same, and I haven’t even bought any converters or storage, which would be required for the large-scale application of solar energy. And if the reader doesn’t believe my quick and dirty calculation, the reader is redirected to the relevant reports by the US Energy and Information Administration[92] and to Figure S – 6 of this 2014 study commissioned by the European Commission,[93] which support my conclusion that though the initial investment sum of nuclear energy is high, the costs per unit of electricity generated over the lifetime of a nuclear power plant is in fact comparable to other sources of electricity.
In his second article, comrade Ford quotes some numbers supposedly showing that nuclear energy is “more than three times as expensive as the cheapest source of energy”. However, when we look at his source it becomes clear that he has conveniently left out the storage capacity required for such large scale application of variable energy sources. Nor has he even considered the costs of the required adjustments of the electricity grid to support more decentralized sources. Comrade Ford continues by reminding us that we should not forget other renewables such as “wave power, hydroelectricity, radiant energy, biomass, etc, etc.”, and I of course agree with those first two. However, I’m very curious about what the comrade means with ‘radiant energy’ because to me that just sounds exactly like solar energy. And I am downright shocked that the comrade would mention biomass in this list, since the carbon-dioxide-equivalent emissions of biomass are only marginally better than coal as we have seen in part one. Biomass can only be considered renewable if it only uses biomass as fuel that is waste anyway, which is very often not the case, and even if it is you’re better off using the wood to make furniture in my opinion.
Further on in his second article, comrade Ford highlights the enormous increase in the share of wind energy in the UK over the past 10 years, from 3% to 25%, impressive indeed. However, when comrade Ford states that “when it has been exceptionally blowy, we have even had a situation where wind power accounted for 59.9% of electricity generated in Britain”, he misses an important point. A single type of energy source more than doubling its output on certain days is in fact a problem for the stability of the electricity grid. To accommodate this increase, the energy output somewhere else has to decrease, or the excess energy should be stored. At the moment the former is almost always the case since massive storage infrastructure is currently not in place, gas and other power plants have to decrease their output on such days, which in principle is not a bad thing. However, there comes a point where the share of variable energy sources is sufficiently large that electricity storage is an absolute necessity to prevent the overall input from exceeding the output, which would cause a power outage (too much electricity is in fact just as problematic as too little). And this is why massive investments in electricity storage are now suddenly becoming an immediate concern, the alternative would be to add a replacement scalable constant energy source to keep the grid stable: nuclear energy. With his example, comrade Ford tries to convince us that we can use wind energy to power society, but instead he highlights how very close we are to reaching this critical share of variable energy sources where the lack of investments in massive storage infrastructure would suddenly become a very real problem.
“We could go into the exact whys and wherefores of it all” comrade Ford states, thinking that presenting some numbers with pound signs in front of them is enough to settle the issue. The ‘why’ of the matter is in fact very important, because there are reasons other than the initial investment sum, that prevent firms from investing in a nuclear power plant. Examples are, the relatively long period it takes before such an investment starts paying off, the public opposition such a company would have to deal with, and the fact that a change in government policy could mean the early closure of the nuclear power plant, in which case the investment might not pay off at all. The fact of the matter is that nuclear energy is not an attractive investment opportunity, which is why it is expensive, and why it is not becoming cheaper as fast as solar and wind. That does not mean that governments should just let the capitalist market decide on the strategy to combat climate change, because as has been proven time and time again this market is not rational, nor does it make decisions that are in the best interests of all of humanity. Our strategy to combat climate change should be based on science, not on what is or is not a guaranteed lucrative investment for some capitalists.
We need as an absolute imperative to transition away from coal and oil - which actually can be done very quickly using existing technologies, especially renewables. Solar and wind are becoming dramatically cheaper, making them perfectly realistic options. But, like fusion, the new and better fission technology being developed right now - even if it works brilliantly - might arrive too late to save the day. Time is running out, as the planet is getting warmer and warmer by the year.
Comrade Ford correctly notes that time is running out, which is why it is all the more surprising that he continues to advocate for a strategy that is already proving to be too slow. We are already behind,[25] and such an observation is usually a reason to reevaluate our approach to the problem and ask ourselves how we can solve the problem faster and more efficiently. Instead comrade Ford suggests we simply keep doing what we’ve been doing and hope that this strategy will somehow speed up, even though one would expect that increasing the share of variable energy sources will only become more and more difficult the larger this share becomes because more and more storage capacity will be required. I do not know where comrade Ford gets the notion that the transition away from coal “can be done very quickly using existing technologies, especially renewables” considering that so far this strategy has proven to be too slow, too bad he did not provide us with a source for this claim.
It is interesting to note that in the first part of his second article comrade Ford dismisses nuclear energy because it would be too expensive under capitalism, he states that:
But back to the price. Communists, of course, have our own way of approaching things - planning and calculating labour hours. But we are highlighting price because this is how capitalism establishes its rationality - through the law of value. An indirect way of working things out, yes, but nonetheless it is the best way capitalism has to tell what is efficient and what is inefficient.
So in essence comrade Ford dismisses nuclear energy because he claims that capitalism tells us that it would be inefficient. However, later on in that very same article, when talking about putting solar panels in the Sahara Desert, comrade Ford says:
If capitalism cannot do that because of national divisions, state breakdown, profit maximisation, the narrow self-interests of the oil majors, etc, then socialism would have every interest in taking up such a project on a global scale. Vladimir Lenin and the Bolsheviks presided over the highly successful Goelro plan. We in our turn shall preside over Desertec 4.0. Workers’ power plus solar power equals global sustainability.
And all of a sudden comrade Ford has completely reversed his position, we apparently do not have to take capitalism into account at all for comrade Ford’s grand plans of a massive solar farm in the Sahara Desert. Though I admire comrade Ford’s optimism, I would not dismiss the notion of Saharan solar energy as a possibility in a socialist future. We obviously cannot expect that the socialist revolution will take place before climate catastrophe hits, so we will have to deal with the problem of climate change within a capitalist context. Furthermore, it seems to me that, given a capitalist framework, it would be a lot easier for a government to build a couple of nuclear power plants even if the capitalists are hesitant to invest, than it would be to realize comrade Ford’s dream of Saharan solar energy, which would require the collaboration of multiple countries in a region that, as comrade Ford admits, is considered ‘politically unstable’.
Lastly, I completely reject comrade Jacobs’s absurd dichotomous choice between climate warming or nuclear power, runaway climate change or a thousand Sizewell C’s. The fact of the matter is that if you thought there was just about to be a qualitative tilt in the climate pattern which would fry the planet, the last thing you would rationally do is choose the nuclear option - given its dangers, cost and how long it takes to put it into operation.
And there is comrade Ford’s final argument: it would take too long to build a nuclear power plant. For starters the energy transition is already taking too long, the strategy is already too slow, and we are already behind schedule. What this means is that we need to diversify our approach to the energy transition, we need to heavily invest in all non-fossil energy sources, not just solar and wind. We need to embrace nuclear energy, lest we risk getting slowed down even further by the bottleneck that is electricity storage. It is precisely short-term thinking that got us into this fossil-mess in the first place, so please let’s not use the same short-term thinking now. Yes it would take relatively long to build a nuclear power plant, and yes we might miss the 2030 goals, but we are going to miss those goals anyway. So we can either stay on the current path, miss the 2030 goals, and in 2030 we would still be behind schedule for the 2050 goals. Or we can start investing in nuclear energy now, in which case we would probably also miss the 2030 goals, but we would make a major leap forward between 2031 and 2033 when the nuclear power plants that we’ve been building become operational. And then there would still be some hope of meeting the 2050 goals.
Conclusion
In order to effectively combat climate change and to create the most stable and robust electricity grid we should diversify our approach, solely wind and solar energy is not enough, nuclear energy can and should play a role in this diversified energy transition. Nuclear energy is an entire field of many different designs and ideas, it is not a monolithic entity, it is not inherently dangerous, nor is it inherently linked to nuclear weapons. Therefore, we should combat and break the stigma surrounding nuclear energy, so that there might still be some hope to avert complete climate catastrophe.Liked it? Take a second to support Cosmonaut on Patreon! At Cosmonaut Magazine we strive to create a culture of open debate and discussion. Please write to us at submissions@cosmonautmag.com if you have any criticism or commentary you would like to have published in our letters section.
- https://climateclock.net/ ↩
- https://en.wikipedia.org/wiki/Shockley%E2%80%93Queisser_limit ↩
- A. Richter, M. Hermle and S. W. Glunz, Reassessment of the Limiting Efficiency for Crystalline Silicon Solar Cells, in IEEE Journal of Photovoltaics, volume: 3 pages: 1184-1191, October 2013. ↩
- S. Rühle, Tabulated values of the Shockley–Queisser limit for single junction solar cells, in Solar Energy, volume: 130 pages: 139-147, June 2016. ↩
- https://en.wikipedia.org/wiki/Gallium_arsenide#Solar_cells_and_detectors ↩
- https://en.wikipedia.org/wiki/Solar_cell_efficiency ↩
- https://en.wikipedia.org/wiki/Multi-junction_solar_cell ↩
- https://en.wikipedia.org/wiki/Perovskite_solar_cell ↩
- J.F. Geisz, R.M. France, K.L. Schulte, et al., Six-junction III–V solar cells with 47.1% conversion efficiency under 143 Suns concentration, in Nature Energy, volume: 5 pages: 326–335, April 2020. ↩
- K. Bódis, I. Kougias, A. Jäger-Waldau, N. Taylor, and S. Szabó, A high-resolution geospatial assessment of the rooftop solar photovoltaic potential in the European Union, in Renewable and Sustainable Energy Reviews, volume: 114 pages, October 2019. ↩
- https://www.eurobserv-er.org/photovoltaic-barometer-2020/ ↩
- C. Bay Hasager, L. Rasmussen, A. Peña, L. E. Jensen, and P. Réthoré, Wind Farm Wake: The Horns Rev Photo Case, in Energies, volume: 6 pages: 696-716, February 2013. ↩
- https://en.wikipedia.org/wiki/Betz%27s_law ↩
- X. Han, D. Liu, C. Xu, W. Zhong Shen, Atmospheric stability and topography effects on wind turbine performance and wake properties in complex terrain, in Renewable Energy, volume: 126 pages: 640-651, October 2018. ↩
- https://en.wikipedia.org/wiki/Wind_power ↩
- R. J. Barthelmie, and L. E. Jensen, Evaluation of wind farm efficiency and wind turbine wakes at the Nysted offshore wind farm, in Wind Energy, volume: 13 pages: 573–586, June 2010. ↩
- Hasager, et al. ↩
- https://www.energy.eu/publications/a07.pdf ↩
- N. G. Nygaard, and S. D. Hansen, Wake effects between two neighbouring windfarms, in Journal of Physics: Conference Series, volume: 753 pages: 032020, September 2016. ↩
- https://en.wikipedia.org/wiki/Wind_power_in_the_European_Union ↩
- https://en.wikipedia.org/wiki/Grid_energy_storage#Forms ↩
- https://en.wikipedia.org/wiki/Energy_conversion_efficiency ↩
- F. Cebulla, J. Haas, J. Eichman, W. Nowak, and P. Mancarella, How much electrical energy storage do we need? A synthesis for the U.S., Europe, and Germany, in Journal of Cleaner Production, volume: 181 pages: 449-459, April 2018. ↩
- https://www.metabolic.nl/publication/metal-demand-for-renewable-electricity-generation-in-the-netherlands/ ↩
- https://climateactiontracker.org/ ↩
- https://ec.europa.eu/eurostat/statistics-explained/index.php/Energy_statistics_-_an_overview#Gross_inland_energy_consumption ↩
- E. Zorn, and T. R. Walter , Influence of volcanic tephra on photovoltaic (PV)-modules: an experimental study with application to the 2010 Eyjafjallajökull eruption, Iceland, in Journal of Applied Volcanology, volume: 5 pages: 2, January 2016. ↩
- P.A. Kharecha and J.E. Hansen, Prevented Mortality and Greenhouse Gas Emissions from Historical and Projected Nuclear Power, in Environmental Science & Technology, volume: 47 pages: 4889−4895, 2013. ↩
- Ibid. ↩
- https://en.wikipedia.org/wiki/Hydroelectricity ↩
- https://en.wikipedia.org/wiki/Geothermal_power ↩
- https://en.wikipedia.org/wiki/Tidal_power ↩
- https://en.wikipedia.org/wiki/Wave_power ↩
- https://en.wikipedia.org/wiki/Nuclear_power ↩
- https://en.wikipedia.org/wiki/Fr%C3%A9d%C3%A9ric_Joliot-Curie ↩
- https://en.wikipedia.org/wiki/Nuclear_fission ↩
- https://en.wikipedia.org/wiki/Ir%C3%A8ne_Joliot-Curie ↩
- https://en.wikipedia.org/wiki/German_nuclear_weapons_program ↩
- https://en.wikipedia.org/wiki/Law_for_the_Restoration_of_the_Professional_Civil_Service ↩
- https://en.wikipedia.org/wiki/Deutsche_Physik ↩
- https://en.wikipedia.org/wiki/Einstein%E2%80%93Szil%C3%A1rd_letter ↩
- https://en.wikisource.org/wiki/Albert_Einstein_to_Franklin_D._Roosevelt_-_August_2,_1939 ↩
- https://en.wikipedia.org/wiki/Hanford_Site ↩
- https://en.wikipedia.org/wiki/Manhattan_Project ↩
- http://members.peak.org/~danneng/decision/usnews.html ↩
- https://en.wikipedia.org/wiki/Nuclear_arms_race ↩
- https://en.wikipedia.org/wiki/Nuclear_meltdown ↩
- https://en.wikipedia.org/wiki/Actinium ↩
- https://en.wikipedia.org/wiki/Uranium ↩
- https://en.wikipedia.org/wiki/Thorium ↩
- https://en.wikipedia.org/wiki/Actinide ↩
- https://en.wikipedia.org/wiki/Fertile_material ↩
- https://en.wikipedia.org/wiki/Enriched_uranium ↩
- https://en.wikipedia.org/wiki/Thorium_fuel_cycle ↩
- https://en.wikipedia.org/wiki/Plutonium ↩
- https://en.wikipedia.org/wiki/Plutonium-239 ↩
- https://en.wikipedia.org/wiki/Critical_mass ↩
- https://en.wikipedia.org/wiki/Uranium-233 ↩
- https://en.wikipedia.org/wiki/Nuclear_reactor ↩
- https://en.wikipedia.org/wiki/Thorium-based_nuclear_power ↩
- https://en.wikipedia.org/wiki/Molten-Salt_Reactor_Experiment ↩
- https://en.wikipedia.org/wiki/Nuclear_reactor_coolant#Water ↩
- https://en.wikipedia.org/wiki/Liquid_fluoride_thorium_reactor ↩
- https://en.wikipedia.org/wiki/Fusion_power ↩
- https://en.wikipedia.org/wiki/Thermonuclear_weapon ↩
- https://en.wikipedia.org/wiki/Tsar_Bomba ↩
- https://www.bbc.com/future/article/20170816-the-monster-atomic-bomb-that-was-too-big-to-use ↩
- https://en.wikipedia.org/wiki/ITER ↩
- https://en.wikipedia.org/wiki/Alvin_M._Weinberg ↩
- https://en.wikipedia.org/wiki/Nuclear_reactor#Reactor_types ↩
- https://en.wikipedia.org/wiki/Generation_IV_reactor ↩
- https://en.wikipedia.org/wiki/Kyshtym_disaster ↩
- https://en.wikipedia.org/wiki/Chernobyl_disaster ↩
- https://en.wikipedia.org/wiki/RBMK ↩
- https://en.wikipedia.org/wiki/Scram ↩
- http://www.ecolo.org/documents/documents_in_english/cherno-alexander_yuvchenko.htm ↩
- https://en.wikipedia.org/wiki/Chernobyl_Exclusion_Zone ↩
- https://en.wikipedia.org/wiki/Boiling_water_reactor ↩
- https://en.wikipedia.org/wiki/Pressurized_water_reactor ↩
- https://en.wikipedia.org/wiki/Fukushima_Daiichi_nuclear_disaster ↩
- https://en.wikipedia.org/wiki/High-level_radioactive_waste_management ↩
- https://en.wikipedia.org/wiki/Nuclear_reprocessing ↩
- https://en.wikipedia.org/wiki/Radioactive_waste ↩
- https://en.wikipedia.org/wiki/Onkalo_spent_nuclear_fuel_repository ↩
- https://weeklyworker.co.uk/worker/1335/swords-into-ploughshares/ ↩
- https://weeklyworker.co.uk/worker/1337/expensive-dangerous-unnecessary/ ↩
- https://weeklyworker.co.uk/worker/1336/luddite-delusions/ ↩
- https://en.wikipedia.org/wiki/List_of_states_with_nuclear_weapons ↩
- https://atomicinsights.com/how-did-leaders-of-the-hydrocarbon-establishment-build-the-foundation-for-radiation-fears/ ↩
- https://en.wikipedia.org/wiki/Anti-nuclear_movement ↩
- https://en.wikipedia.org/wiki/Radiophobia ↩
- https://www.eia.gov/outlooks/aeo/electricity_generation.php ↩
- https://ec.europa.eu/energy/sites/ener/files/documents/ECOFYS%202014%20Subsidies%20and%20costs%20of%20EU%20energy_11_Nov.pdf ↩